The lensless Bio-FlatScope is a compact, low-cost camera that can detect biological activity that conventional devices cannot detect. The technology could someday be used to detect cancer or sepsis, or it could become a valuable endoscopy tool.
Do you want to listen in on a tiger’s mind while it runs? First and foremost, capture the tiger. Then connect Bio-FlatScope, Rice University’s most recent lensless microscope iteration. According to Jacob Robinson, an electrical and computer engineer at Rice’s George R. Brown School of Engineering who spearheaded the recent effort to test Bio-FlatScope in living organisms, that particular purpose is imaginative but not far-fetched.
The study team’s FlatCam, a lensless device that directs light through a mask and onto a camera sensor, was primarily directed at the outside world. The raw photographs were static, but a special algorithm exploited the data included in them to reconstruct what the camera observed.
The new technology looks inward to scan micron-scale targets inside the body, such as cells and blood veins, even through the skin. Bio-FlatScope can view images that no lensed camera can, such as dynamic changes in fluorescent-tagged neurons in running mice.
The Bio-FlatScope phase mask resembles a random map of a natural landscape, as there are no straight lines. We had to start from scratch and figure out how to make it work in a true biological setting.
Jacob Robinson
One advantage of Bio-FlatScope over conventional microscopes is that light captured by it can be refocused after the fact to expose 3D details. Without lenses, the field of vision of the scope is the size of the sensor (at close proximity to the target) or wider, with no distortion.
According to Robinson, who worked on the technology with colleagues from Rice’s Neuroengineering Initiative, a compact, low-cost Bio-FlatScope could potentially scan for indicators of cancer or sepsis or become a beneficial tool for endoscopy.
The team’s proof-of-concept work also captured images of plants, hydra, and, to a lesser extent, a human. Their findings were published in the journal Nature Biomedical Engineering. According to the researchers, the process combines a complex phase mask to generate light patterns that fall directly onto the semiconductor. The mask of the original FlatCam resembles a bar code and limits the amount of light that reaches the camera sensor. However, it does not perform well with biological samples.
The Bio-FlatScope phase mask resembles a random map of a natural landscape, as there are no straight lines. “We had to start from scratch and figure out how to make it work in a true biological setting,” Robinson explained.
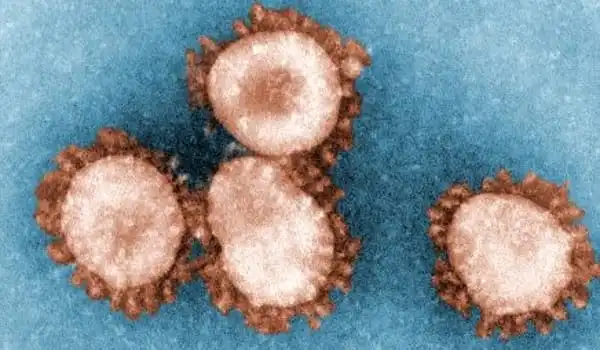
“Being random allows the mask to be quite diversified in gathering light from various directions,” said one of the study’s four lead authors, postdoctoral researcher Vivek Boominathan. “And then we take the random input, which is known as Perlin noise, and process it to create these high-contrast contours.”
Light that passes through the mask appears as a point spread function at the sensor, a pair of hazy blobs that appear useless but are essential for gathering details about things below the diffraction limit that are too small for many microscopes to observe. The size, shape, and distance between the blobs show how far the subject is from the focal plane. Software reinterprets the data into an image that can be refocused at will.
A tiger was out of the question, so the researchers started small, capturing cellular structures in a lily of the valley and then calcium activity in a tiny, jellyfishlike hydra. They moved on to monitoring a running mouse, connecting the Bio-FlatScope to the rodent’s head and placing it on a wheel. The research showed fluorescent-tagged neurons in a region of the animal’s brain, linking activity in the motor cortex with motion and resolving blood capillaries as fine as 10 microns in diameter.
The researchers discovered vascular imaging as the potential clinical use of the Bio-FlatScope in partnership with Rebecca Richards-Kortum and research scientist Jennifer Carns from Rice Bioengineering. Jimin Wu, a graduate student, and co-lead author, provided her lower lip to check if light passing through may reveal structural characteristics of the blood veins within.
“It was a bit of an engineering problem since it’s tough to position the Bio-FlatScope and maintain it there,” Wu explained. “However, it demonstrated that it could be a useful tool for detecting indications of sepsis since pre-sepsis affects the density of the vasculature. Cancer also causes changes in the morphology of the microvasculature.”
“We would think it would be difficult to insert a microscope in that position,” Robinson said, “but perhaps a little clip you put on your lip would be able to look for things like sepsis or malignancies in the oral mucosa.”
Long term, the team envisions a camera that can curve around its target, such as brain tissue, “so it can mimic the morphology of what you’re looking at,” Robinson said. “Alternatively, you could fold it up, stick it in position, and let it unroll and deploy.” “You could also do pretty interesting things by bending it for a fisheye look, or by curving it inward and having extremely great light-collection efficiency,” he explained.
Jesse Adams, a Rice alumnus, and Dong Yan, an Applied Physics graduate student, are among the study’s co-lead authors. Rice graduate students Sibo Gao and Soonyoung Kim are co-authors, as with postdoctoral alumnus Alex Rodriguez, Caleb Kemere, an assistant professor of electrical and computer engineering and bioengineering, and Ashok Veeraraghavan, a professor of electrical and computer engineering.
Richards-Kortum is a Malcolm Gillis University Professor, bioengineering and electrical and computer engineering professor, and the director of the Rice360 Institute for Global Health Technology. Robinson is an associate professor of electrical and computer engineering as well as bioengineering, and he, together with Kemere and Veeraraghavan, is a key member of the Rice Neuroengineering Initiative.