If the petrochemical sector is to ever wean itself off oil and gas, it must identify sustainably sourced chemicals that can be seamlessly integrated into existing processes for producing fuels, lubricants, and polymers.
The apparent solution is to make such molecules biologically, but microbial products differ from fossil fuel hydrocarbons in two important ways: they contain too much oxygen and have too many other atoms dangling off the carbons.
Microbial hydrocarbons must often be de-oxygenated, reduced, and stripped of superfluous chemical groups in order to work in existing synthesis methods, all of which consume energy.
A hydrocarbon is a type of organic substance that only contains hydrogen and carbon atoms. They are group 14 hybrids, which means they contain hydrogen as well as carbon, silicon, germanium, tin, and lead atoms from the carbon 14 group.
Microbes have been designed by a team of scientists from the University of California, Berkeley, and the University of Minnesota to produce hydrocarbon chains that can be deoxygenated more readily and with less energy, using only the sugar glucose that the bacteria consume and a little heat.
The approach enables the microbial manufacture of a wide range of compounds that are currently made from oil and gas, including lubricants derived from medium-chain hydrocarbons, which have between eight and ten carbon atoms in their chain.
Almost all hydrocarbons, such as petroleum and natural gas, are found naturally in crude oils. Crude oil is high in hydrogen and carbon atoms since it is made up of degraded organic materials.
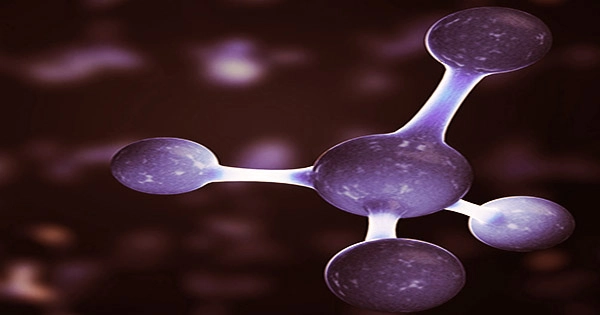
They’re also found in a variety of trees and plants, and they combine to make carotene, a natural pigment found in carrots and green leaves. The majority of natural crude rubber (98%) is made up of a hydrocarbon polymer, which is generated when a chainlike molecule with numerous units connected together occurs.
“Part of the issue with trying to move to something like glucose as a feedstock for making molecules or to drive the chemical industry is that the fossil fuel structures of petrochemicals are so different they’re usually fully reduced, with no oxygen substitutions,” said Michelle Chang, UC Berkeley professor of chemistry and of chemical and biomolecular engineering.
“Bacteria know how to make all these complex molecules that have all these functional groups sticking out from them, like all-natural products, but making petrochemicals that we’re used to using as precursors for the chemical industry is a bit of a challenge for them.”
“This process is one step towards deoxygenating these microbial products, and it allows us to start making things that can replace petrochemicals, using just glucose from plant biomass, which is more sustainable and renewable,” she said. “That way we can get away from petrochemicals and other fossil fuels.”
Part of the issue with trying to move to something like glucose as a feedstock for making molecules or to drive the chemical industry is that the fossil fuel structures of petrochemicals are so different they’re usually fully reduced, with no oxygen substitutions.
Michelle Chang
Others have devised microbial techniques for building shorter and longer chains, up to roughly 20 carbons, but the bacteria were programmed to generate medium-length hydrocarbon chains, which has never been done before.
However, Chang claims that the process may easily be altered to generate chains of different lengths, such as short-chain hydrocarbons used as precursors to the most common polymers, such as polyethylene. Her findings were published in the journal Nature Chemistry this week by her and her colleagues.
A bioprocess to make olefins
Simple linear chains of carbon atoms with a hydrogen atom connected to each carbon make up fossil hydrocarbons. However, microbially generated precursors that are oxygenated and have carbon atoms adorned with many additional atoms and tiny molecules cannot simply be substituted by chemical procedures geared for turning these into high-value products.
Chang and her team, which included former UC Berkeley postdoctoral researchers Zhen Wang and Heng Song as co-first authors, searched databases for enzymes that might synthesize medium-chain hydrocarbons in order to induce bacteria to generate something that could replace these fossil fuel precursors. They were also looking for an enzyme that could add a unique chemical group called carboxylic acid to one end of a hydrocarbon, converting it to a fatty acid.
Overall, the researchers forced E. coli bacteria to ferment glucose and create the necessary medium-chain fatty acid by inserting five different genes into the bacteria. The additional enzymatic reactions were independent of or orthogonal to the bacteria’s existing enzyme pathways, which worked better than tinkering with the bacteria’s complicated metabolic network.
“We identified new enzymes that could actually make these mid-size hydrocarbon chains and that were orthogonal, so separate from fatty acid biosynthesis by the bacteria. That allows us to run it separately, and it uses less energy than it would if you use the native synthase pathway,” Chang said. “The cells consume enough glucose to survive, but then alongside that, you have your pathway chewing through all the sugar to get higher conversions and a high yield.”
The final step of creating a medium-chain fatty acid primed the product for easy conversion to olefins, which are precursors to polymers and lubricants, via a catalytic reaction.
The UC Berkeley team collaborated with a Minnesota group led by Paul Dauenhauer, who demonstrated that removing the carboxylic acid from the final microbial products 3-hydroxyoctanoic and 3-hydroxydecanoic acids using a simple acid-based catalytic reaction known as Lewis acid catalysis (named after UC Berkeley chemist Gilbert Newton Lewis) produced the olefins heptene and nonene, respectively.
Lewis acid catalysis consumes a fraction of the energy required by redox reactions to remove oxygen from natural products and produce pure hydrocarbons.
“The biorenewable molecules that Professor Chang’s group made were perfect raw materials for catalytic refining,” said Dauenhauer, who refers to these precursor molecules as bio-petroleum.
“These molecules contained just enough oxygen that we could readily convert them to larger, more useful molecules using metal nanoparticle catalysts. This allowed us to tune the distribution of molecular products as needed, just like conventional petroleum products, except this time we were using renewable resources.”
Heptene, which has seven carbons, and nonene, which has nine, can be used as lubricants directly, or they can be cracked down to smaller hydrocarbons and used as precursors to plastic polymers like polyethylene or polypropylene, or they can be linked together to form even longer hydrocarbons like those found in waxes and diesel fuel.
“This is a general process for making target compounds, no matter what chain length they are,” Chang said. “And you don’t have to engineer an enzyme system every time you want to change a functional group or the chain length or how branched it is.”
Despite their metabolic engineering feat, Chang stated that the long-term and more sustainable goal would be to completely redesign processes for synthesizing industrial hydrocarbons, such as plastics, so that they are optimized to use the types of chemicals that microbes normally produce, rather than modifying microbial products to fit into existing synthetic processes.
“There’s a lot of interest in the question, ‘What if we look at entirely new polymer structures?’,” she said. “Can we make monomers from glucose by fermentation for plastics with similar properties to the plastics that we use today, but not the same structures as polyethylene or polypropylene, which are not easy to recycle.”
The Center for Sustainable Polymers, a National Science Foundation-funded Center for Chemical Innovation, helped fund the research (CHE-1901635). Edward Koleski, Noritaka Hara, and Yejin Min of UC Berkeley, as well as Dae Sung Park and Gaurav Kumar of the University of Minnesota, are co-authors.