Quantum computing relies on the properties of quantum mechanics, which are different from classical mechanics, to perform certain types of computation. One important property of quantum computing is the ability to perform certain operations on a quantum state, such as quantum gates, that would be impossible to perform classically. Another key feature of quantum computing is the ability to operate on a superposition of states, which allows for the simultaneous manipulation of multiple states at once.
In terms of thermodynamics, one of the main challenges facing quantum computing is the issue of decoherence, which is the process by which a quantum state becomes mixed with its environment and loses its coherence. This can occur due to interactions with the environment, such as thermal noise, and can cause errors in quantum computations. As a result, efforts are being made to develop techniques to mitigate decoherence and preserve the coherence of quantum states, such as error-correcting codes and quantum error correction.
Another area of interest in the thermodynamics of quantum computing is the study of the energy requirements of quantum computing. Quantum computing requires a large amount of energy to perform operations and maintain the coherence of quantum states, and this energy consumption has been a topic of research. However, the energy requirements of quantum computing are still an open question and more research is needed to fully understand the thermodynamics of quantum computing.
One aspect of quantum computer research that has largely gone unnoticed until now is the generation of heat. Physicists are now focusing on heat as an interference factor, and they have developed a method for measuring the heat generated by a superconducting quantum system experimentally.
Our trick is to have the resistor measuring the temperature inside a resonator – an oscillating circuit – that produces a strong response at a certain frequency. This resonator oscillates at 600 megahertz and can be read out very quickly.
Wolfgang Belzig
Heat and computers do not get along. When computers overheat, they perform poorly or even crash. But what about the future quantum computers? These high-performance devices are even more heat sensitive. This is due to the fact that their fundamental computational units — quantum bits or “qubits” — are based on highly sensitive units, some of which are individual atoms, and heat can be a significant interference factor.
The basic dilemma: In order to retrieve the information of a qubit, its quantum state must be destroyed. The heat released in the process can interfere with the sensitive quantum system. The quantum computer’s own heat generation could consequently become a problem, suspect physicists Wolfgang Belzig (University of Konstanz), Clemens Winkelmann (Néel Institute, Grenoble), and Jukka Pekola (Aalto University, Helsinki). In experiments, the researchers have now documented the heat generated by superconducting quantum systems. To do so, they developed a method that can measure and display the temperature curve to one-millionth of a second throughout the process of reading one qubit. “This means we are monitoring the process as it takes place,” says Wolfgang Belzig. The method was recently published in the journal Nature Physics.
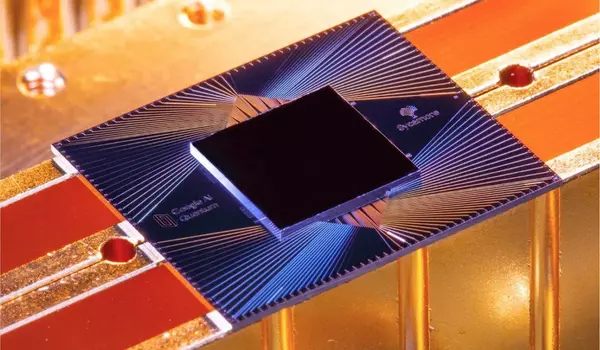
Superconducting quantum systems produce heat
Until now, research on quantum computing has focused on the basics of getting these high-performance computers to work: Much research mainly involves the coupling of quantum bits and identifying which material systems are optimal for qubits. Little consideration has been given to heat generation: Especially in the case of superconducting qubits constructed using a supposedly ideal conducting material, researchers have often assumed that no heat is generated or that the amount is negligible. “That is simply not true,” Wolfgang Belzig says and adds: “People often think of quantum computers as idealized systems. However, even the circuitry of a superconducting quantum system produces heat.” How much heat, is what the researchers can now measure precisely.
A thermometer for the quantum bit
The measurement method was developed for superconducting quantum systems. These systems are based on superconducting circuits that use “Josephson junctions” as a central electronic element. “We measure the electron temperature based on the conductivity of such contacts. This is nothing special in and of itself: Many electronic thermometers are based in some way on measuring conductivity using a resistor. The only problem is: How quickly can you take the measurements?” Clemens Winkelmann explains. Changes to a quantum state take only a millionth of a second.
“Our trick is to have the resistor measuring the temperature inside a resonator – an oscillating circuit – that produces a strong response at a certain frequency. This resonator oscillates at 600 megahertz and can be read out very quickly,” Winkelmann explains.
Heat is always generated
With their experimental evidence, the researchers want to draw attention to the thermodynamic processes of a quantum system. “Our message to the quantum computing world is: Be careful and watch out for heat generation. We can even measure the exact amount,” Winkelmann adds.
This heat generation could become particularly relevant for scaling up quantum systems. Wolfgang Belzig explains: “One of the greatest advantages of superconducting qubits is that they are so large because this size makes them easy to build and control. On the other hand, this can be a disadvantage if you want to put many qubits on a chip. Developers need to take into account that more heat will be produced as a result and that the system needs to be cooled adequately.”