Chemists have been looking for effective catalysts for converting methane to methanol. Adding water to the reaction can help with some problems, but it also complicates the process. Now, a team has identified a new approach that uses a common industrial catalyst to effectively complete the conversion both with and without water. The findings suggest strategies for improving water-free conversion catalysts.
Chemists have been looking for efficient catalysts to convert methane, a major component of plentiful natural gas, into methanol, an easily transported liquid fuel and building block for the production of other valuable chemicals. Adding water to the reaction can help with some problems, but it also complicates the process. Now, a team at the U.S. Department of Energy’s Brookhaven National Laboratory has discovered a new method that uses a common industrial catalyst to complete the conversion effectively both with and without water. The findings, which were published in the Journal of the American Chemical Society, suggest strategies for improving water-free conversion catalysts.
“Water is a trick that has been used for a long time to get this reaction going and it definitely helps. It improves selectivity and aids in the extraction of methanol” José Rodriguez, a Brookhaven Lab Catalysis Group leader who also holds an adjunct appointment at Stony Brook University (SBU) in the departments of Chemistry, Materials Science, and Chemical Engineering explained.
As demonstrated in a recent related study by this group, adding water prevents the reaction from overheating and converting the desired product, methanol, into carbon monoxide (CO) and carbon dioxide (CO2) (CO2). However, adding water complicates and increases the cost. Furthermore, at the temperatures and quantities required for this reaction, the water exists as large amounts of steam, which would have to be controlled in an industrial setting.
So the Brookhaven team set out to see if they could run the reaction without using water by changing the catalyst, which is the substance that brings the reactants together and guides them along a specific reaction pathway.
Water is a trick that has been used for a long time to get this reaction going and it definitely helps. It improves selectivity and aids in the extraction of methanol. Copper-zinc oxide has the highest selectivity of any catalyst tested for this reaction without the addition of water – around 30%.
José Rodriguez
Catalytic conversion
The new paper describes how a common copper-zinc oxide catalyst can direct the reaction along different pathways depending on the presence of water. “Copper-zinc oxide is a commercial catalyst that is readily available and reasonably priced,” said one of the study’s co-authors, Sanjaya Senanayake. “We wanted to see if it could be used for methane-to-methanol conversion.”
According to the findings of their study, copper-zinc oxide has the highest selectivity of any catalyst tested for this reaction without the addition of water – around 30%. That is, when the reaction is run without water, methanol, the desired product (rather than CO or CO2), accounts for 30% of the products. (When run with water, the copper-zinc oxide catalyst produced methanol with 80 percent selectivity.) In comparison, the team’s previous studies of this reaction using a cerium oxide catalyst yielded almost no methanol in the absence of water.
“Overoxidation – the transformation of the methanol into carbon monoxide and carbon dioxide, is one of the major challenges of this methanol synthesis reaction in the presence of only methane and oxygen,” said study co-author Ping Liu. She mentioned how previous research on the cerium catalyst revealed how water helped to prevent overoxidation by removing the produced methanol before it could be further transformed.
The team conducted detailed studies using a variety of techniques that worked hand-in-hand with theoretical calculations to reveal crucial details of the reaction mechanism to discover how the copper-zinc catalyst achieves 80 and 30 percent specificity with and without water, respectively.
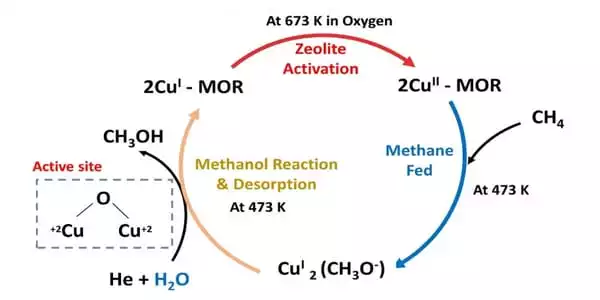
X-ray studies
“Two of our SBU graduate students, Ivan Orozco and Feng Zhang, and one of our postdocs, Zongyuan Liu, collaborated with Slavomr Nemák, a long-time collaborator, at the Advanced Light Source (ALS) – a DOE Office of Science user facility at Lawrence Berkeley National Laboratory – to find evidence of methanol formation on the surface of the catalyst,” Senanayake explained. “The technique, known as ‘ambient-pressure x-ray photoemission spectroscopy (XPS),’ employs the ALS’s bright x-ray beams to detect carbon, hydrogen, oxygen, and metal-oxygen combinations at the active sites of the catalyst as the reaction occurs.”
The samples were studied under various reaction conditions by the scientists. They varied the concentrations of methane, oxygen, and water (including no water), as well as the pressure and temperature, to determine which chemical species were present at various stages of the reaction.
“Because each compound has its own ‘chemical fingerprint,’ we can see how these reactants are transformed into intermediates and final products under different conditions,” Rodriguez explained. The XPS fingerprints clearly demonstrated the formation of methanol. However, in order to determine which sites on the catalyst were involved in the reactions, the team used theoretical modeling.
Modeling atomic interactions
The team used a scanning tunneling microscope in Brookhaven’s Chemistry Department to study the atomic-level structure of the catalyst and then used those structural details to build computational models of the atomic arrangements.
“On the surface of the catalyst, there are many different active sites,” Liu explained. Another SBU graduate student, Erwei Huang, and Liu ran “density functional theory” (DFT) calculations and kinetic modeling on computing clusters at Brookhaven Lab’s Center for Functional Nanomaterials (CFN) and the National Energy Research Scientific Computing Center (NERSC) at Lawrence Berkeley National Laboratory to understand those sites and determine whether and how they interacted with the reactants and products.
DFT calculations determine how the reactants (methane, oxygen, and water) evolve as they interact with one another and with the catalyst, as well as how much energy is required to move from one atomic arrangement to the next. Kinetic modeling simulates all of the possible pathways for those transformations to occur under reaction conditions.
The team was able to identify the most energy-efficient (and thus most likely) path for how methane is converted into methanol with and without water using this combination of techniques. The findings included information on which catalytic sites were involved and which intermediates should be present at various stages of the reaction. The team then used “chemical fingerprinting” measurements at ALS to confirm these catalytic interactions and intermediates.
Pathways to improvement
Together, the data indicate that the reaction proceeds along two different pathways involving two different sites of the copper-zinc-oxide catalyst, one for the reaction with water and one for the reaction without water.
“The specific configuration of active sites for the reaction with water differs from the configuration without water, and the mechanism differs as well — it’s practically two different processes,” Rodriguez explained. However, even in the absence of water, “the binding between the methanol and the catalyst is strong enough to allow the methanol to form from methane but weak enough to allow the methanol to come off the surface as a gas before it is further oxidized to CO or CO2,” according to Liu.
“As soon as the methanol enters the gas phase, you can condense it all and separate the liquid methanol,” Rodriguez explained. That rapid “desorption” of methanol from the catalyst surface, which prevents the methanol from reacting further with oxygen, also eliminates a potentially explosive step. The team is already looking for ways to improve the catalyst based on their new understanding of the reaction mechanisms. Their goal is to achieve at least 60-70 percent selectivity without the use of water.
“The atomic-level understanding is far superior to anything we’ve ever had. We know atom by atom that copper-zinc oxide is far superior for the preferred no-water reaction condition “Senanayake explained. DFT calculations and kinetic modeling will be used to test other compositions in the next step, with the goal of improving methane conversion and methanol selectivity even further.
“We’ll use the theory to narrow down the candidates based on the mechanistic understanding gained from previous research,” Liu explained. “The experimentalists will then conduct synthesis and characterization studies to determine whether these other compositions will work.”