In the face of rising CO2 levels, scientists are looking for sustainable methods of removing carbon dioxide from the atmosphere, known as direct air capture. A novel form of porous material, a covalent organic framework (COF) with linked amines, stands out for its long-lasting properties and excellent CO2 adsorption and desorption at low temperatures. The material would be compatible with carbon capture technologies currently utilized for point source capture.
Capturing and storing the carbon dioxide humans make is critical to lowering atmospheric greenhouse gasses and delaying global warming, but current carbon capture methods are only effective for concentrated sources of carbon, such as power plant emissions. The same methods cannot efficiently capture carbon dioxide from ambient air, where concentrations are hundreds of times lower than in flue gases.
However, direct air capture, or DAC, is expected to halt the rise in CO2 levels, which have reached 426 parts per million (ppm), 50% higher than before the Industrial Revolution. According to the Intergovernmental Panel on Climate Change, without it, humanity will fail to meet its goal of keeping warming to 1.5 °C (2.7 °F) above pre-existing world averages.
A new form of absorbent material discovered by chemists at the University of California, Berkeley, may help the globe achieve negative emissions. The porous material, a covalent organic framework (COF), absorbs CO2 from the atmosphere without being degraded by water or other impurities, which is one of the limits of current DAC technologies.
We took a powder of this material, put it in a tube, and we passed Berkeley air – just outdoor air – into the material to see how it would perform, and it was beautiful. It cleaned the air entirely of CO2.
Omar Yaghi
“We took a powder of this material, put it in a tube, and we passed Berkeley air – just outdoor air – into the material to see how it would perform, and it was beautiful. It cleaned the air entirely of CO2. Everything,” said Omar Yaghi, the James and Neeltje Tretter Professor of Chemistry at UC Berkeley and senior author of a paper that will appear online in the journal Nature.
“I am excited about it because there’s nothing like it out there in terms of performance. It breaks new ground in our efforts to address the climate problem,” he added.
According to Yaghi, the new material could be substituted easily into carbon capture systems already deployed or being piloted to remove CO2 from refinery emissions and capture atmospheric CO2 for storage underground. UC Berkeley graduate student Zihui Zhou, the paper’s first author, said that a mere 200 grams of the material, a bit less than half a pound, can take up as much CO2 in a year — 20 kilograms (44 pounds) — as a tree.
“Flue gas capture is a way to slow down climate change because you are trying not to release CO2 to the air. Direct air capture is a method to take us back to like it was 100 or more years ago,” Zhou said. “Currently, the CO2 concentration in the atmosphere is more than 420 ppm, but that will increase to maybe 500 or 550 before we fully develop and employ flue gas capture. So if we want to decrease the concentration and go back to maybe 400 or 300 ppm, we have to use direct air capture.”
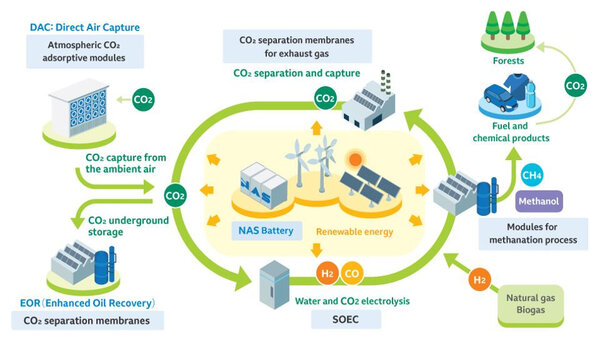
COF vs MOF
Yaghi is the inventor of COFs and MOFs (metal-organic frameworks), both of which are rigid crystalline structures with regularly spaced internal pores that provide a large surface area for gases to stick or adsorb. Some MOFs that he and his lab have developed can adsorb water from the air, even in arid conditions, and when heated, release the water for drinking. He has been working on MOFs to capture carbon since the 1990s, long before DAC was on most people’s radar screens, he said.
His lab developed MOF-808, a very promising CO2 adsorbent, two years ago, but the researchers discovered that the MOFs broke down after hundreds of cycles of adsorption and desorption. These MOFs were internally decorated with amines (NH2 groups), which effectively bind CO2 and are a common component of carbon capture materials. In fact, the most common carbon capture method is bubbling exhaust gases through liquid amines, which collect the carbon dioxide. Yaghi did, however, observe that the energy-intensive regeneration and instability of liquid amines impede their future industrialization.
Working with collaborators, Yaghi found why some MOFs degrade for DAC applications: they are unstable under basic rather than acidic environments, and amines are bases. He and Zhou collaborated with colleagues in Germany and Chicago to develop a stronger substance called COF-999. MOFs are kept together by metal atoms, whereas COFs are bound together by covalent carbon-carbon and carbon-nitrogen double bonds, which are among the strongest chemical bonds found in nature.
COF-999 pores, like MOF-808, are adorned with amines on the interior, enabling for the uptake of additional CO2 molecules.
“Trapping CO2 from air is a very challenging problem,” Yaghi said. “It’s energetically demanding, you need a material that has high carbon dioxide capacity, that’s highly selective, that’s water stable, oxidatively stable, recyclable. It needs to have a low regeneration temperature and needs to be scalable. It’s a tall order for a material. And in general, what has been deployed as of today are amine solutions, which are energy intensive because they’re based on having amines in water, and water requires a lot of energy to heat up, or solid materials that ultimately degrade with time.”
Yaghi and his team have spent the last 20 years developing COFs that have a strong enough backbone to withstand contaminants, ranging from acids and bases to water, sulfur and nitrogen, that degrade other porous solid materials. The COF-999 is assembled from a backbone of olefin polymers with an amine group attached. Once the porous material has formed, it is flushed with more amines that attach to NH2 and form short amine polymers inside the pores. Each amine can capture about one CO2 molecule.
When 400 ppm CO2 air is fed through the COF at ambient temperature (25 °C) and 50% humidity, it takes roughly 18 minutes to reach half capacity and two hours to fill. However, this is dependent on the sample form and can be accelerated to a fraction of a minute when tuned. Heating to a relatively moderate temperature of 60 °C (140 °F) releases the CO2, and the COF is ready to adsorb CO2 again. It outperforms other solid sorbents by holding up to 2 millimoles of CO2 per gram.
Yaghi pointed out that not all of the amines in the interior polyamine chains are now capturing CO2, thus it may be able to increase the pores to bind more than double the amount.
“This COF has a strong chemically and thermally stable backbone, it requires less energy, and we have shown it can withstand 100 cycles with no loss of capacity. No other material has been shown to perform like that,” Yaghi said. “It’s basically the best material out there for direct air capture.”
Yaghi believes that artificial intelligence can help accelerate the development of even better COFs and MOFs for carbon capture and other applications, particularly by finding the chemical conditions required to synthesis their crystalline structures. He is the scientific director of the Bakar Institute of Digital Materials for the Planet (BIDMaP), a UC Berkeley research lab that uses artificial intelligence to create cost-effective, easily deployable versions of MOFs and COFs to help minimize and address the effects of climate change.