Scientists have discovered how lattice vibrations and spins communicate with each other in an electromagnon, a hybrid excitation. To accomplish this, they used a novel combination of experiments at the SwissFEL X-ray free electron laser. Understanding this fundamental process at the atomic level paves the way for ultrafast magnetic control with light.
Particles and their various properties cooperate in wave-like motions known as collective excitations within the atomic lattice of a solid. A phonon is the collective excitation of atoms in a lattice when they jiggle together. A magnon is formed when the atomic spins – the magnetization of the atoms – move together.
The situation becomes more complicated. Some of these collective excitations communicate with one another in what are known as hybrid excitations. An electromagnon is one type of hybrid excitation. In contrast to conventional magnons, electromagnons get their name from their ability to excite atomic spins using an electric field of light: an exciting prospect for a wide range of technical applications. However, their atomic secret life is not well understood.
Measuring the phononic part alone at Bernina was a significant step forward. Accessing magnetic motion with Furka is an experimental possibility that exists almost nowhere else in the world.
Urs Staub
It has been hypothesized that during an electromagnon, the atoms in the lattice wiggle, and the spins wobble in an excitation that is essentially a phonon and a magnon combined. However, only the spin motion has ever been measured since they were first proposed in 2006. How the atoms within the lattice move – if they move at all – has remained a mystery. So too has an understanding of how the two components talk to each other.
Now, in a sophisticated series of experiments at the Swiss X-ray free-electron laser SwissFEL, researchers at PSI have added these missing pieces to the jigsaw. “With a better understanding of how these hybrid excitations work, we can now start to look into opportunities to manipulate magnetism on an ultrafast timescale,” explains Urs Staub, head of the Microscopy and Magnetism Group at PSI, who led the study.
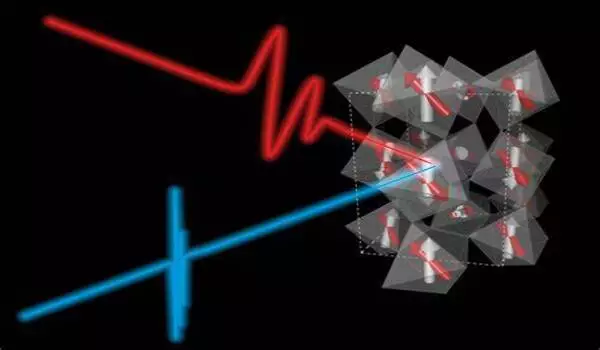
First the atoms, then the spins
In their experiments at SwissFEL, the researchers used a terahertz laser pulse to induce an electromagnon in a crystal of multiferroic hexaferrite. They then used time-resolved X-ray diffraction experiments to capture ultrafast snapshots of how the atoms and spins moved in response to the excitation. They demonstrated both that the atoms within the lattice move in an electromagnon and how energy is transferred between the lattice and the spin.
Their research found that the atoms move first, followed by the spins fractionally later. When the terahertz pulse strikes the crystal, the electric field pushes the atoms into motion, triggering the phononic part of the electromagnon. This motion generates an effective magnetic field, which moves the spins.
“Our experiments revealed that the excitation does not move the spins directly. It was previously unclear whether this would be the case,” explains Hiroki Ueda, beamline scientist at SwissFEL and the first author of the publication.
Going further, the team could also quantify how much energy the phononic component acquires from the terahertz pulse and how much energy the magnonic component acquires through the lattice. “This is an important piece of information for future applications in which one seeks to drive the magnetic system,” adds Ueda.
One free electron laser, two beamline, two crystal modes
Key to their discovery was the ability to measure both the atomic motions and the spins in complementary time-resolved X-ray diffraction experiments at the hard and soft X-ray beamlines of SwissFEL.
Using hard X-rays at the Bernina experimental station, the team studied the motion of atoms within the lattice. The recently developed set-up of the experimental station including specially designed sample chambers allows unique ultrafast measurements using terahertz fields in solids at very low temperatures.
The team used soft X-rays, which are more sensitive to changes in magnetic systems, to study the motion of the spins. These experiments were carried out at the Furka experimental station, which has recently been made available to the public.They could focus specifically on the signal from the spins by tuning the X-ray energy to a resonance in the material – information that is normally masked.
“Measuring the phononic part alone at Bernina was a significant step forward. Accessing magnetic motion with Furka is an experimental possibility that exists almost nowhere else in the world,” Staub says.