A new study explains why certain attempts to convert carbon dioxide into fuel have failed and suggests viable alternatives. If researchers could develop a technique to chemically transform carbon dioxide into fuels or other items, they may make a significant dent in greenhouse gas emissions. However, many such processes that were promising in the lab have not worked as predicted in scaled-up formats suitable for usage with a power plant or other emission sources.
MIT researchers have now identified, quantified, and modeled a primary cause of poor performance in such conversion systems. The culprit is a local depletion of carbon dioxide gas just adjacent to the electrodes used to catalyze the conversion. The scientists discovered that just pulsing the current off and on at precise intervals can relieve the problem by allowing time for the gas to build back up to the required amounts adjacent to the electrode.
The findings, which could lead to the development of new materials and designs for electrochemical carbon dioxide conversion systems, were published in the journal Langmuir in a paper co-authored by MIT postdoc lvaro Moreno Soto, graduate student Jack Lake, and mechanical engineering professor Kripa Varanasi.
“I believe that carbon dioxide mitigation is one of the most critical concerns of our time,” Varanasi says. While much of the research in the area has focused on carbon capture and sequestration, which involves pumping the gas into a deep underground reservoir or converting it to an inert solid such as limestone, another promising avenue has been converting the gas into other carbon compounds such as methane or ethanol, which can be used as fuel, or ethylene, which can be used as a precursor to useful polymers.
Thermal techniques necessitate extremely high temperatures and do not provide high-value chemical compounds, which are a barrier for light-activated processes as well, efficiency is always in play, and it is always a concern.
Professor Kripa Varanasi
Such conversions can be accomplished in a variety of ways, including electrochemical, thermocatalytic, photothermal, or photochemical processes. “Each of these has its own set of concerns or challenges,” Varanasi says. Thermal techniques necessitate extremely high temperatures and do not provide high-value chemical compounds, which is a barrier for light-activated processes as well, he claims. “Efficiency is always in play, and it is always a concern.”
The team has concentrated on electrochemical techniques with the goal of producing “higher-C products” – molecules with more carbon atoms that tend to be higher-value fuels due to their energy per weight or volume. The most difficult difficulty in these processes has been to limit competing reactions that can occur at the same time, particularly the splitting of water molecules into oxygen and hydrogen.
The reactions take occur as a stream of liquid electrolytes containing dissolved carbon dioxide passes over an electrically charged metal catalytic surface. However, once the carbon dioxide is transformed, it leaves a region in the electrolyte stream where it has essentially been used up, and the reaction inside this depleted zone shifts to water splitting instead. The researchers discovered that this undesired reaction consumes energy and significantly decreases the overall efficiency of the conversion process.
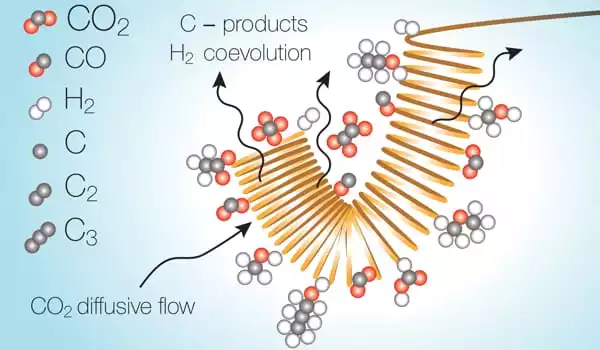
“There are a number of organizations working on this, as well as a number of catalysts out there,” says Varanasi. “I believe hydrogen co-evolution will become a bottleneck in all of these.”
A pulsed system, they discovered, can overcome this depletion by simply shutting off the voltage, stopping the reaction, allowing the carbon dioxide to diffuse back into the depleted zone and reach useable levels, and then restarting the reaction.
According to the researchers, many times, groups have discovered promising catalyst materials but haven’t performed their lab studies long enough to see these depletion effects, leaving them disappointed when attempting to scale up their systems. Furthermore, the compounds produced are determined by the concentration of carbon dioxide near the catalyst. As a result, depletion might alter the product mix and render the process unstable. “If you want to design a system that works at an industrial scale, you have to be able to run things over a long period of time and not have these types of effects that affect the efficiency or reliability of the process,” Varanasi adds.
The researchers investigated three different catalyst materials, including copper, and “really focused on ensuring that we understood and could quantify the depletion effects,” Lake adds. They were able to devise a simple and dependable method of monitoring the efficiency of the conversion process as it occurred by detecting the changing pH levels, a measure of acidity, in the system’s electrolyte.
They used more sophisticated analytical instruments to characterize reaction products in their testing, such as gas chromatography for gaseous products analysis and nuclear magnetic resonance characterization for the system’s liquid products. However, their investigation revealed that a simple pH measurement of the electrolyte close to the electrode during operation may offer an adequate assessment of the reaction’s efficiency as it progressed.
According to Moreno Soto, the capacity to quickly monitor the reaction in real-time could eventually lead to a system optimized by machine-learning approaches, managing the synthesis rate of the desired compounds through constant feedback.
Now that the process has been defined and quantified, the researchers believe that alternative measures to mitigate carbon dioxide depletion may be devised and simply tested using their methodologies.
According to Lake, this research demonstrates that “no matter what your catalyst material is” in such an electrocatalytic system, “you’ll be affected by this problem.” And now, according to the model they created, it’s easy to identify exactly what sort of time window needs to be examined in order to obtain an accurate sense of the material’s overall efficiency, as well as what type of system operations could enhance its efficacy.