Graphene is a carbon allotrope made up of a single layer of atoms organized in a two-dimensional honeycomb lattice nanostructure. The name is derived from “graphite” and the suffix -ene, showing that the graphite allotrope of carbon contains a large number of double bonds.
Because high-quality graphite has excellent mechanical strength, thermal stability, high flexibility, and extremely high in-plane thermal and electric conductivities, it is one of the most important advanced materials used in a wide range of applications, including light thermal conductors in cell phones. Highly Ordered Pyrolytic Graphite (HOPG), for example, is one of the most commonly used laboratory materials.
These excellent properties originate from the layered structure of graphite, where the strong covalent binding between carbon atoms in a graphene layer contribute to the excellent mechanical properties. Thermal and electric conductivities and the very weak interaction between graphene layers leads to the high flexibility of graphite.
This success really on a few critical issues of the experimental design: (1) the successful synthesis of large size single crystalline Ni films serves as an ultra-flat substrate and thus the disorders in the synthesized graphite can be avoided; (2) the isothermal growth of 100,000 graphene layers over ~ 100 hours allows every graphene layer be synthesized under exact same chemical environment and temperature thus ensure the uniformity of the graphite quality; (3) continuous carbon feeding through the back side of the Ni foil allows the contiguous growth of graphene layers in a very large growth rate, ~ one layer per five seconds.
Professor Ding
Despite the fact that graphite has been known in its natural form for over 1,000 years and its artificial synthesis has been studied for over 100 years, the quality of graphite samples, both natural and synthesized, is far from ideal. In graphitic materials, the largest single crystalline graphite domains are typically less than 1 mm in size, which contrasts sharply with the size of many crystals, such as quartz single crystals and silicon single crystals, which can reach meter scale.
The very small size of single crystalline graphite is due to the weak interaction between graphite layers, which makes maintaining the flatness of a graphene layer difficult during the growth process. Therefore graphite can be easily broken into a few single crystals with disordered grain boundaries.
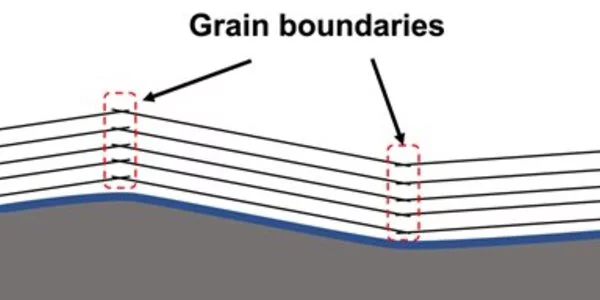
To solve this critical issue, a Distinguished Professor of Ulsan National Institute of Science and Technology (UNIST) and his collaborators, Professor Kaihui Liu, Professor Enge Wang of Peking University and others have proposed a strategy to synthesize single-crystalline graphite films orders of magnitude large, up to inch scale. In their approach, single crystalline Ni foils are used as a substrate, and caron atoms are supplied from the back side of the Ni foils through an isothermal dissolution-diffusion-precipitation process.
Instead of using gas phase carton source, they choose solid carbon materials to feed the graphite growth. The new strategy creates ~1 inch single crystalline graphite films of 35 μm thick, or more than 100,000 graphene layers, within a few days. The single crystalline graphite has the recorded thermal conductivity of ~2,880 Wm-1K-1, negligible impurity contents and smallest layer distances compared with all available graphite samples.
“This success really on a few critical issues of the experimental design: (1) the successful synthesis of large size single crystalline Ni films serves as an ultra-flat substrate and thus the disorders in the synthesized graphite can be avoided; (2) the isothermal growth of 100,000 graphene layers over ~ 100 hours allows every graphene layer be synthesized under exact same chemical environment and temperature thus ensure the uniformity of the graphite quality; (3) continuous carbon feeding through the back side of the Ni foil allows the contiguous growth of graphene layers in a very large growth rate, ~ one layer per five seconds,” Professor Ding explained.
The findings of this research were published in the October 2022 issue of Nature Nanotechnology.