The solid-electrolyte interphase, a chemical layer in batteries that is critical to the stability of lithium metal anodes, was studied using high-energy x-rays. Chemists unraveled this complex chemical mechanism that is crucial for boosting energy density.
The term “electronic commerce” refers to the sale of goods and services over the internet. Batteries are at the heart of society’s most critical green innovations, from electric vehicles to renewable grid-scale energy storage but they need to pack more energy to make these technologies widespread and practical.
Now, a team of scientists led by chemists at the U.S. Department of Energy’s (DOE) Brookhaven National Laboratory and Pacific Northwest National Laboratory (PNNL) has unraveled the complex chemical mechanisms of a battery component that is crucial for boosting energy density: the interphase. Their work published today in Nature Nanotechnology.
DOE’s Battery500 consortium zeroes-in on lithium metal anodes
Many electronic devices, including smartphones and electric vehicles, are currently powered by traditional lithium-ion batteries. While lithium-ion batteries have grown in popularity due to their high efficiency and long lifespan, they face challenges in more demanding applications, such as powering electric vehicles over long distances.
To build a better battery for electric vehicles, researchers across several national laboratories and DOE-sponsored universities have formed a consortium called Battery500. The consortium, led by PNNL, aims to produce battery cells with an energy density of 500 watt-hours per kilogram, which is more than double the energy density of today’s state-of-the-art batteries. To that end, the team is concentrating on lithium metal batteries. While graphite anodes are used in lithium-ion batteries, lithium metal anodes are used in these batteries.
The interphase affects the overall cyclability of the battery. It’s a critical but elusive system. Many techniques have the potential to harm this small, sensitive sample, which also has crystalline and amorphous phases.
Enyuan Hu
Lithium metal anodes provide a much higher energy density than graphite anodes, but there are trade-offs. One of the biggest challenges scientists currently face is finding a way to stabilize the anode as the battery charges and discharges.
Brookhaven Lab and PNNL scientists led an in-depth study on the solid-electrolyte interphase of lithium metal batteries in search of such a method. As a battery charges and discharges, a chemical layer forms between the anode and the electrolyte. Scientists have learned that the interphase is the key to stabilizing lithium metal batteries, but it is a very sensitive sample with convoluted chemistry, making it difficult to study and, therefore, difficult to fully understand.
“The interphase affects the overall cyclability of the battery. It’s a critical but elusive system “Brookhaven chemist Enyuan Hu, who led the study, explained. “Many techniques have the potential to harm this small, sensitive sample, which also has crystalline and amorphous phases.”
The scientific community has conducted many studies using a variety of experimental techniques, including cryo-electron microscopy, to better understand the interphase but the picture is still far from being clear and complete.
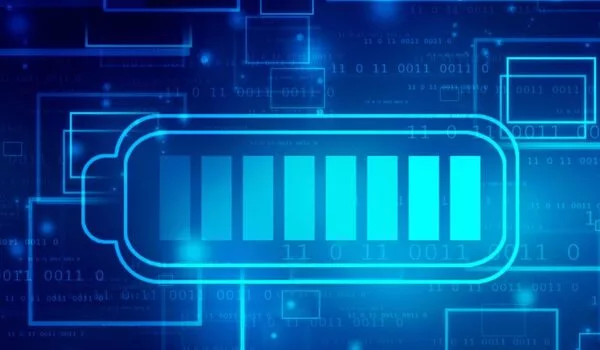
“A comprehensive understanding of the interphase lays the groundwork for building an effective interphase,” said PNNL scientist Xia Cao, who co-led the study and led the electrolyte development. “The Battery500 Consortium strongly encourages collaborations. We have worked closely with Brookhaven Lab on many scientific projects, particularly understanding the interphase.”
The team used a one-of-a-kind tool called the National Synchrotron Light Source II to delve deeper into the complex and elusive chemistry of the interphase (NSLS-II).
NSLS-II shines light on interphase chemistry
NSLS-II is a DOE Office of Science User Facility at Brookhaven Lab that generates ultrabright x-rays for studying the atomic-scale makeup of materials. Hu and colleagues have been leveraging the advanced capabilities of the X-ray Powder Diffraction (XPD) beamline at NSLS-II to make new discoveries in battery chemistry for many years. Building on their previous successes, the team returned to XPD to gather their most precise findings on the interphase yet.
“We’ve previously discovered that high energy synchrotron x-rays do not damage the interphase sample,” Hu said. “This is critical because one of the most difficult aspects of characterizing the interphase is that the samples are extremely sensitive to other types of radiation, including low-energy x-rays. So in this work, we took advantage of two techniques that use high energy x-rays, x-ray diffraction and pair distribution function analysis, to capture the chemistries of both the crystalline and the amorphous phases in the lithium metal anode interphase.”
After cycling a lithium metal battery 50 times and harvesting enough interphase sample, the team disassembled the cell, scraped off a trace amount of interphase powder from the surface of the lithium metal, and directed XPD’s high energy x-rays at the sample to reveal its convoluted chemistry.
“XPD is one of the few beamlines in the world that is capable of carrying out this research,” said Sanjit Ghose, lead beamline scientist at XPD and a co-author of the study. “The beamline provided three advantages for this work: a small absorption cross-section, which damages the sample less; combined techniques, x-ray diffraction to get the phase information and pair distribution function for real space information; and a high-intensity beam for delivering quality data from a trace sample.”
This unique combination of advanced x-ray techniques provided the team with a detailed chemical map of the interphase components – their origins, functionalities, interactions, and evolutions.
“We concentrated on three different components of the interphase,” said Brookhaven postdoc Sha Tan, the paper’s first author. “First was lithium hydride and its formation mechanism. We previously discovered the presence of lithium hydride in the interphase, and this time we found the source of the hydrogen.”
The team determined that lithium hydroxide, which is naturally present in the lithium metal anode, is the most likely contributor to lithium hydride. Controlling the composition of this compound will allow scientists to create an improved interphase with the highest possible performance.
“Second, we studied lithium fluoride, which is very important for electrochemical performance, and found that it can be formed at a large scale in low-concentration electrolytes,” Tan said.
Previously, scientists believed that lithium fluoride could only be formed in electrolytes with high concentrations of salts. Thus, the work provides evidence that low-concentration electrolytes, which are more cost-effective, can potentially perform well in these battery systems.
“Third, we looked at lithium hydroxide to see how it is consumed during battery cycling. These are all novel discoveries that are critical to understanding the interphase.” These findings, when combined, shed light on previously overlooked interphase components and will enable more accurate and controllable interphase design for lithium metal batteries.