The most advanced brain map to date, that of an insect, has been completed by researchers, a landmark achievement in neuroscience that brings scientists closer to a true understanding of the mechanism of thought.
The international team led by Johns Hopkins University and the University of Cambridge created a breathtakingly detailed diagram tracing every neural connection in the brain of a larval fruit fly, an archetypal scientific model with human-like brains. The findings, which are likely to underpin future brain research and inspire new machine learning architectures, were published today in the journal Science.
“If we want to understand who we are and how we think, part of that is understanding the mechanism of thought,” said senior author Joshua T. Vogelstein, a Johns Hopkins biomedical engineer who specializes in data-driven projects including connectomics, the study of nervous system connections. “And the key to that is knowing how neurons connect with each other.”
If we want to understand who we are and how we think, part of that is understanding the mechanism of thought. And the key to that is knowing how neurons connect with each other.
Joshua T. Vogelstein
The first attempt at mapping a brain resulted in a partial map and a Nobel Prize for a 14-year study of the roundworm that began in the 1970s. Partial connectomes have since been mapped in a variety of systems, including flies, mice, and even humans, but these reconstructions typically only represent a tiny fraction of the total brain. Only a few small species with a few hundred to a few thousand neurons in their bodies have comprehensive connectomes—a roundworm, a larval sea squirt, and a larval marine annelid worm.
This team’s connectome of a baby fruit fly, Drosophila melanogaster larva, is the most complete as well as the most expansive map of an entire insect brain ever completed. It includes 3,016 neurons and every connection between them: 548,000.
“It’s been 50 years and this is the first brain connectome. It’s a flag in the sand that we can do this,” Vogelstein said. “Everything has been working up to this.”
Even with the most advanced modern technology, mapping entire brains is difficult and time-consuming. Obtaining a complete cellular-level picture of a brain necessitates slicing the brain into hundreds or thousands of individual tissue samples, all of which must be imaged with electron microscopes before the laborious process of reconstructing all of those pieces, neuron by neuron, into a complete, accurate portrait of a brain. That took more than a decade with the baby fruit fly. A mouse’s brain is estimated to be a million times larger than that of a baby fruit fly, implying that mapping anything close to a human brain is unlikely in the near future, if not in our lifetimes.
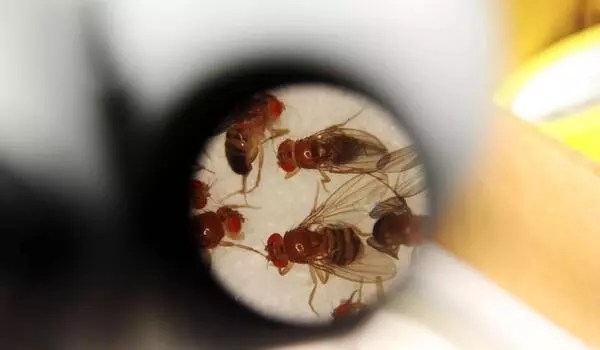
The fruit fly larva was chosen on purpose because, for an insect, it shares much of its fundamental biology with humans, including a comparable genetic foundation. It also exhibits complex learning and decision-making abilities, making it a valuable model organism in neuroscience. In addition, its relatively small brain can be imaged and its circuits reconstructed in a reasonable amount of time.
Nonetheless, it took the University of Cambridge and Johns Hopkins 12 years to complete the work. Imaging each neuron took about a day. The high-resolution images of the brain were created by Cambridge researchers and manually studied to find individual neurons, rigorously tracing each one and connecting their synaptic connections.
Cambridge forwarded the data to Johns Hopkins, where the team spent more than three years analyzing the brain’s connectivity using original code they created. The Johns Hopkins team devised methods for identifying groups of neurons based on shared connectivity patterns, and then investigated how information could spread through the brain.
Finally, the entire team charted every neuron and every connection, and classified each neuron based on its function in the brain. They discovered that the busiest circuits in the brain were those that led to and from neurons in the learning center.
The methods developed by Johns Hopkins are applicable to any brain connection project, and their code is available to anyone attempting to map an even larger animal brain, according to Vogelstein, who added that despite the challenges, scientists are expected to tackle the mouse within the next decade. Other groups are already at work on mapping the adult fruit fly brain. Co-first author Benjamin Pedigo, a Johns Hopkins doctoral candidate in Biomedical Engineering, believes the team’s code will help reveal important differences between adult and larval brain connections. Pedigo expects their analysis techniques to lead to a better understanding of variations in brain wiring as more larvae and other related species’ connectomes are generated.
The circuit features of the fruit fly larva work were strikingly similar to prominent and powerful machine learning architectures. The team anticipates that further research will reveal even more computational principles and may inspire new artificial intelligence systems.
“What we learned about fruit fly code will have implications for human code,” Vogelstein said. “That’s what we’re looking for: how to write a program that leads to a human brain network.”