A protein has been genetically engineered to emit the shortest-wavelength fluorescence light reported to date. They achieved this by optimizing the interactions between the fluorescence center (chromophore) and its surroundings in a novel way compared to previous reports. The resulting fluorescence emission was bright and stable across a useful pH range. This research will benefit both basic and applied research, such as understanding the behavior of healthy and diseased cells.
Consider how difficult it would be to visually keep track of five people scattered throughout a stadium. Researchers accomplish far more amazing feats by simultaneously tracking multiple cellular factors, but to advance current capabilities, they require an expanded fluorescence toolkit.
Now, in a study recently published in Communications Biology, researchers from SANKEN (The Institute of Scientific and Industrial Research) at Osaka University have genetically modified a protein to exhibit the shortest fluorescence emission wavelength currently available.
The short-emission wavelength limit of fluorescent proteins has stayed the same for the past 10 years. This is because previous researchers have usually focused on making minor changes to one of the amino acids of green fluorescent protein mutants.
Kazunori Sugiura
Fluorescence is a popular method for microscopically observing the inner workings of cells. A biomolecule of interest, for example, can be genetically modified to include a fluorescent protein (i.e., a fluorophore) that emits a specific color (i.e., wavelength) of light. By combining various types of biomolecules with different fluorophores, each emitting a different wavelength of light, it is possible to identify and track all of these different biomolecules at the same time. Extending the range of possible emitted wavelengths can increase the number of biomolecules that can be tracked at the same time. This is the issue that the researchers set out to solve.
“The short-emission wavelength limit of fluorescent proteins has stayed the same for the past 10 years,” explains Kazunori Sugiura, lead author. “This is because previous researchers have usually focused on making minor changes to one of the amino acids of green fluorescent protein mutants.”
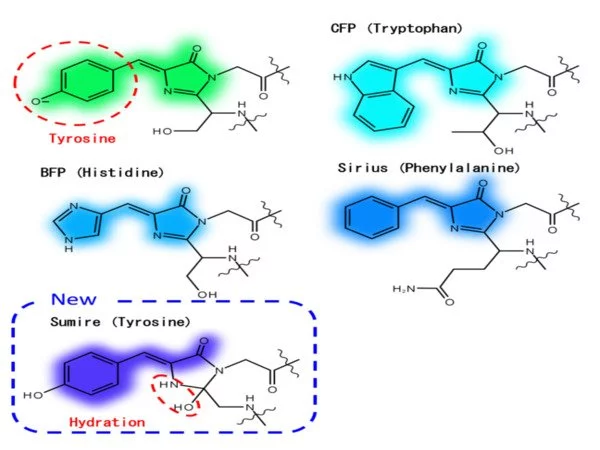
Instead, the researchers at Osaka University concentrated on optimizing the interactions between the fluorescence center (i.e., the chromophore) and the surrounding water molecules and amino acids. The resulting fluorophore, Sumire, exhibited several notable fluorescence properties: (1) emission at 414 nanometers, a new record; (2) brightness nearly four times that of the state-of-the-art; and (3) stable emission from pH 5.5-9.0, which encompasses the majority of the pH range seen in most cells.
“We also achieved fluorescence resonance energy transfer, a common biomolecular imaging technique, between Sumire and common commercial protein fluorophores,” says Takeharu Nagai, senior author. “This further illustrates the compatibility of Sumire with modern multi-parameter analysis.”
This study was successful in using genetic engineering to expand the cellular imaging toolkit by modifying the chromophore of a fluorescent protein in an unexpected way. The approach developed by Osaka University researchers will be useful for further expanding the range of attainable fluorescence wavelengths from engineered proteins, allowing researchers to uncover biological principles important in normal health and disease.
Because of the rapid advances in fluorescent protein and synthetic fluorophore technology, an increasing number of studies are using live-cell imaging techniques to provide critical insight into the fundamental nature of cellular and tissue function. As a result, live-cell imaging has become a required analytical tool in most cell biology laboratories, as well as a routine methodology used in the diverse fields of neurobiology, developmental biology, pharmacology, and many other related biomedical research disciplines.