Several technology companies, including Google, Microsoft, and IBM, have made significant investments in quantum computing systems based on microwave superconducting circuit platforms in order to scale them up from small research-oriented systems to commercialized computing platforms in recent years. However, realizing the full potential of quantum computers necessitates a significant increase in the number of qubits, the quantum computer’s building blocks that can store and manipulate quantum information.
The scientists used a lithium niobate electro-optical phase modulator and optical fibers instead of HEMT amplifiers and coaxial cables. At cryogenic temperatures, microwave signals from superconducting circuits modulate a laser carrier and encode information on the output light. Optical fibers are approximately 100 times more effective heat isolators than coaxial cables and 100 times more compact. This enables the design of large-scale quantum systems without the need for massive cryogenic cooling power. Furthermore, direct conversion of microwave signals to the optical domain allows for long-distance transfer and networking between quantum systems.
Leading technology companies such as Google, Microsoft, and IBM, have been investing heavily in quantum computing systems based on microwave superconducting circuit platforms and have been looking to scale them in order to develop commercial computing platforms.
A successful quantum computer necessitates a large number of qubits, the quantum computer’s building blocks, in order to store and manipulate quantum information. However, thermal noise generated by electron movement can contaminate quantum signals. To avoid this, superconducting quantum systems must operate at ultra-low temperatures (less than 20 millikelvins), which cryogenic helium-dilution refrigerators can achieve.
At low temperatures, the output microwave signals of such systems are amplified by low-noise high-electron-mobility transistors (HEMTs). Signals are then routed outside the refrigerator via microwave coaxial cables, which are the simplest way to control and read superconducting devices but are poor heat isolators and take up a lot of space, which becomes a problem when we need to scale up qubits into the thousands.
Professor Tobias J. Kippenberg’s group at EPFL’s School of Basic Sciences has now developed a novel approach for reading out superconducting circuits using light, overcoming the scaling challenges of quantum systems. The work is published in Nature Electronics.
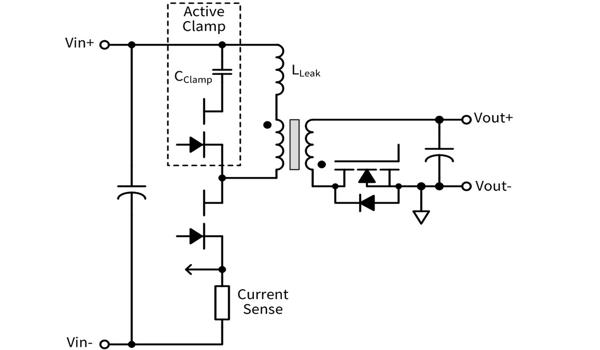
The scientists used a lithium niobate electro-optical phase modulator and optical fibers instead of HEMT amplifiers and coaxial cables. At cryogenic temperatures, microwave signals from superconducting circuits modulate a laser carrier and encode information on the output light. Optical fibers are approximately 100 times more effective heat isolators than coaxial cables and 100 times more compact. This enables the design of large-scale quantum systems without the need for massive cryogenic cooling power. Furthermore, direct conversion of microwave signals to the optical domain allows for long-distance transfer and networking between quantum systems.
To validate this method, the researchers conducted conventional coherent and incoherent spectroscopic measurements on a superconducting electromechanical circuit, which revealed perfect agreement between optical and traditional HEMT measurements. At low temperatures, the output microwave signals of such systems are amplified by low-noise high-electron-mobility transistors (HEMTs). The scientists used a lithium niobate electro-optical phase modulator and optical fibers instead of HEMT amplifiers and coaxial cables.
“We show a proof-of-principle experiment using a novel optical readout protocol to optically measure a superconducting device at cryogenic temperatures,” says Amir Youssefi, a Ph.D. student on the project. “It paves the way for future quantum systems to be scaled.” To validate this method, the researchers conducted conventional coherent and incoherent spectroscopic measurements on a superconducting electromechanical circuit, which revealed perfect agreement between optical and traditional HEMT measurements.
Although this project used a commercial electro-optical phase modulator, the researchers are currently working on advanced electro-optical devices based on integrated lithium niobate technology to significantly improve the conversion efficiency and noise of their method.