Microelectronics can be used to control and monitor the behavior of biological robots, also known as bio-bots. These devices are typically composed of living cells that have been genetically modified or engineered to respond to specific stimuli, such as electrical signals or chemical compounds. By integrating microelectronics into the design of these bio-bots, researchers can remotely control and monitor their movements and actions, allowing for more precise and accurate manipulation of biological systems. This technology has a wide range of potential applications, including in medicine, agriculture, and environmental monitoring.
They started by walking. Then they saw the light. Miniature biological robots now have a new trick: remote control. According to researchers from the University of Illinois Urbana-Champaign, Northwestern University, and other institutions, the hybrid “eBiobots” are the first to combine soft materials, living muscle, and microelectronics. They published their centimeter-scale biological machines in the journal Science Robotics.
“Integrating microelectronics allows the merger of the biological world and the electronics world, both with many advantages of their own, to now produce these electronic biobots and machines that could be useful for many medical, sensing, and environmental applications in the future,” said study co-leader Rashid Bashir, an Illinois professor of bioengineering and dean of the Grainger College of Engineering.
By creating the first hybrid bioelectronic robot, we are paving the way for a new paradigm of applications for health care innovation, such as in-situ biopsies and analysis, minimally invasive surgery, or even cancer detection within the human body.
Zhengwei Li
Bashir’s lab was the first to develop biobots, which are small biological robots powered by mouse muscle tissue grown on a soft 3D-printed polymer skeleton. In 2012, they demonstrated walking biobots, and in 2016, they demonstrated light-activated biobots. The light activation provided the researchers with some control, but practical applications were limited by the issue of how to deliver light pulses to the biobots outside of a lab setting.
Northwestern University professor John A. Rogers, a pioneer in flexible bioelectronics whose team assisted in the integration of tiny wireless microelectronics and battery-free micro-LEDs, provided the answer. This enabled the researchers to control the eBiobots remotely.
“This unusual combination of technology and biology opens up vast opportunities in creating self-healing, learning, evolving, communicating, and self-organizing engineered systems. We feel that it’s a very fertile ground for future research with specific potential applications in biomedicine and environmental monitoring,” said Rogers, a professor of materials science and engineering, biomedical engineering, and neurological surgery at Northwestern University and director of the Querrey Simpson Institute for Bioelectronics.
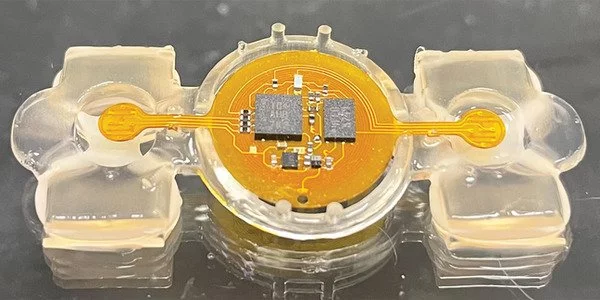
The researchers set out to eliminate bulky batteries and tethering wires in order to give the biobots the freedom of movement required for practical applications. According to co-first author Zhengwei Li, an assistant professor of biomedical engineering at the University of Houston, the eBiobots harvest power and provide a regulated output voltage to power the micro-LEDs.
The researchers can send the eBiobots a wireless signal that causes the LEDs to pulse. The LEDs cause the light-sensitive engineered muscle to contract, causing the polymer legs to move, and causing the machines to “walk.” The micro-LEDs are so precise that they can activate specific muscle groups, causing the eBiobot to turn in the desired direction.
The researchers used computational modeling to improve the robustness, speed, and maneuverability of the eBiobot design and component integration. Mattia Gazzola, an Illinois professor of mechanical sciences and engineering, led the simulation and design of the eBiobots. The scaffolds’ iterative design and additive 3D printing enabled rapid cycles of experiments and performance improvement, according to Gazzola and co-first author Xiaotian Zhang, a postdoctoral researcher in Gazzola’s lab.
According to co-first author Youngdeok Kim, who completed the work as a graduate student at Illinois, the design allows for future integration of additional microelectronics, such as chemical and biological sensors, or 3D-printed scaffold parts for functions like pushing or transporting things that the biobots encounter.
The incorporation of electronic sensors or biological neurons would allow the eBiobots to detect and respond to environmental toxins, biomarkers for disease, and other possibilities, according to the researchers.
“By creating the first hybrid bioelectronic robot, we are paving the way for a new paradigm of applications for health care innovation, such as in-situ biopsies and analysis, minimally invasive surgery, or even cancer detection within the human body,” Li explained.