A team from the United States Department of Energy’s Oak Ridge National Laboratory, Stanford University, and Purdue University created and demonstrated a novel, fully functional quantum local area network, or QLAN, to enable real-time adjustments to information shared with geographically isolated systems at ORNL using entangled photons passing through an optical fiber.
This network exemplifies how experts might routinely connect quantum computers and sensors on a practical scale, realizing the full potential of these next-generation technologies on the path to the much-anticipated quantum internet. The team’s findings, published in PRX Quantum, represent the culmination of years of related research.
Local area networks that connect traditional computing devices are nothing new, and QLANs have been tested successfully in tabletop studies. The most common example of quantum communications in the field thus far has been quantum key distribution, but this procedure is limited because it only establishes security, not entanglement, between sites.
“We’re attempting to lay the groundwork for a quantum internet by understanding critical functions like entanglement distribution bandwidth,” said Nicholas Peters, Quantum Information Science section head at ORNL. “Our goal is to develop the fundamental tools and building blocks required to demonstrate quantum networking applications in order for them to be deployed in real networks to realize quantum advantages.”
A team has developed and demonstrated a novel, fully functional quantum local area network, or QLAN, to enable real-time adjustments to information shared with geographically isolated systems using entangled photons passing through the optical fiber.
When two photons (light particles) are paired together, or entangled, they exhibit quantum correlations that are stronger than those possible using any classical method, regardless of their physical distance. These interactions allow for counterintuitive quantum communications protocols that can only be realized with quantum resources.
Remote state preparation is one such protocol that uses entanglement and classical communications to encode information by measuring one half of an entangled photon pair and effectively converting the other half to the desired quantum state. While earning his doctorate in physics, Peters led the first general experimental realization of remote state preparation in 2005. The team demonstrated the scalability of entanglement-based quantum communications by applying this technique across all of the paired links in the QLAN, which had never been done before on a network.
This method enabled the team to connect three remote nodes known as “Alice,” “Bob,” and “Charlie” – names commonly used for fictional characters who can communicate via quantum transmissions – located in three separate research laboratories on ORNL’s campus. The photons distributed entanglement to Bob and Charlie via ORNL’s existing fiber-optic infrastructure from the laboratory containing Alice and the photon source.
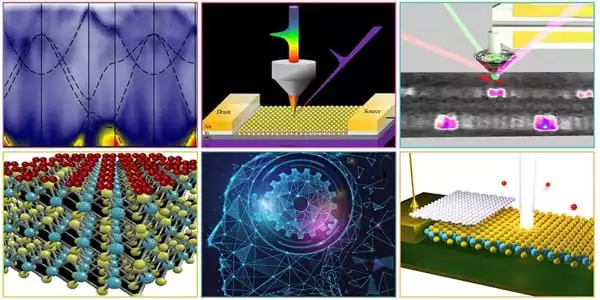
Quantum networks are incompatible with amplifiers and other traditional signal-boosting resources because they interfere with the quantum correlations shared by entangled photons. With this potential disadvantage in mind, the team implemented flexible grid bandwidth provisioning, which employs wavelength-selective switches to assign and reallocate quantum resources to network users without disconnecting the QLAN. This technique provides a type of built-in fault tolerance, allowing network operators to respond to an unexpected event, such as a broken fiber, by rerouting traffic to other areas without disrupting network performance or jeopardizing security protocols.
“Because network demand may change over time or with different configurations, you don’t want to have a system with fixed wavelength channels that always assigns particular users the same portions,” said Joseph Lukens, an ORNL Wigner Fellow and research scientist who also serves as the team’s electrical engineering expert. “Instead, you want the ability to provide more or less bandwidth to network users based on their needs.”
When compared to traditional classical networks, quantum networks require much closer synchronization of each node’s activity. The researchers relied on GPS, the same versatile and cost-effective technology that uses satellite data to provide everyday navigation services, to meet this requirement. The team shared the signal with each node using a GPS antenna in Bob’s laboratory to ensure that the GPS-based clocks were synchronized within a few nanoseconds and would not drift apart during the experiment.
Following the acquisition of precise timestamps for the arrival of entangled photons captured by photon detectors, the team transferred these measurements from the QLAN to a traditional network, where they compiled high-quality data from all three laboratories.
“This part of the project evolved into a difficult classical networking experiment with very tight tolerances,” Lukens explained. “Timing on a traditional network rarely necessitates that level of precision or that much attention to detail in terms of coding and synchronization between laboratories.”
The QLAN demonstration would have produced lower quality data and fidelity, a mathematical metric related to quantum network performance that measures the distance between quantum states if the GPS signal had not been present.
The team anticipates that minor modifications to the QLAN, such as adding more nodes and nesting wavelength-selective switches together, will result in quantum versions of interconnected networks – the literal definition of the internet.
“The internet is a large network composed of many smaller networks,” explained Muneer Alshowkan, an ORNL postdoctoral research associate who contributed valuable computer science expertise to the project. “Connecting the QLAN to other quantum networks is the next big step toward the development of a quantum internet.”
Furthermore, the findings of the team could be used to improve other detection techniques, such as those used to search for evidence of elusive dark matter, the invisible substance thought to be the universe’s primary source of matter.
“Imagine constructing networks of quantum sensors capable of detecting fundamental high-energy physics effects,” Peters said. “By developing this technology, we hope to reduce the sensitivity required to measure those phenomena, which will help with the ongoing search for dark matter and other efforts to better understand the universe.”
The researchers are already planning their next experiment, which will focus on implementing even more advanced timing synchronization methods to reduce the number of accidentals (noise sources in the network) and improve the QLAN’s quality of service even further.