Hydrogen is an enthralling energy carrier. It is made from electricity and water. Its conversion to heat or power is straightforward and clean. When hydrogen reacts with oxygen, it produces water. There are no pollutants produced or emitted. The water is returned to nature in its original location. However, hydrogen, the most abundant chemical element on the planet, does not exist in its pure form in nature. Using only cheap raw materials, a light-activated catalyst efficiently converts ammonia into clean-burning hydrogen.
Rice University researchers created a critical light-activated nanomaterial for the hydrogen economy. A team from Rice University’s Laboratory for Nanophotonics, Syzygy Plasmonics Inc., and Princeton University’s Andlinger Center for Energy and the Environment developed a scalable catalyst that converts ammonia into clean-burning hydrogen fuel using only the power of light.
The study was published today in the journal Science.
The study is a result of government and industry investment in developing infrastructure and markets for carbon-free liquid ammonia fuel that will not contribute to global warming. With one nitrogen and three hydrogen atoms per molecule, liquid ammonia is lightweight and high in energy. The new catalyst converts the molecules into hydrogen gas, a clean-burning fuel, and nitrogen gas, the most abundant component of the Earth’s atmosphere. It also does not require heat, unlike traditional catalysts. It instead obtains energy from light, either sunlight or energy-efficient LEDs.
This discovery paves the way for sustainable, low-cost hydrogen that could be produced locally rather than in massive centralized plants.
Peter Nordlander
The pace of chemical reactions typically increases with temperature, and chemical producers have capitalized on this for more than a century by applying heat on an industrial scale. The burning of fossil fuels to raise the temperature of large reaction vessels by hundreds or thousands of degrees results in an enormous carbon footprint. Chemical producers also spend billions of dollars each year on thermocatalysts – materials that don’t react but further speed reactions under intense heating.
“Transition metals like iron are typically poor thermocatalysts,” said study co-author Naomi Halas of Rice. “This work shows they can be efficient plasmonic photocatalysts. It also demonstrates that photocatalysis can be efficiently performed with inexpensive LED photon sources.”
“This discovery paves the way for sustainable, low-cost hydrogen that could be produced locally rather than in massive centralized plants,” said co-author Peter Nordlander.
Platinum and related precious metals such as palladium, rhodium, and ruthenium are used to make the best thermocatalysts. Halas and Nordlander spent years developing light-activated metal nanoparticles, also known as plasmonic metal nanoparticles. The best are typically made with precious metals such as silver and gold.
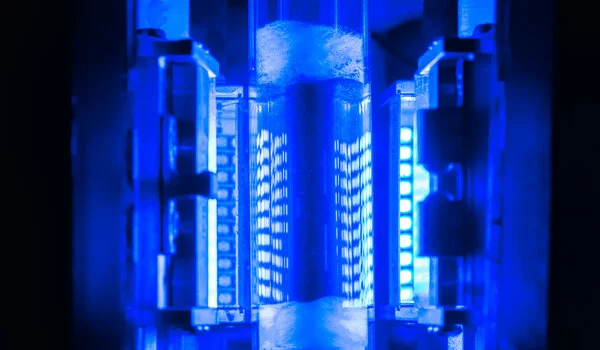
Following their discovery in 2011 of plasmonic particles that emit short-lived, high-energy electrons known as “hot carriers,” they discovered in 2016 that hot-carrier generators could be married with catalytic particles to produce hybrid “antenna-reactors” in which one part harvested energy from light and the other part used the energy to drive chemical reactions with surgical precision.
Halas, Nordlander, their students, and collaborators have worked for years to find non-precious metal alternatives for both the energy-harvesting and reaction-speeding halves of antenna reactors. The new study is a culmination of that work. In it, Halas, Nordlander, Rice alumnus Hossein Robatjazi, Princeton engineer and physical chemist Emily Carter, and others show that antenna-reactor particles made of copper and iron are highly efficient at converting ammonia. The copper, energy-harvesting piece of the particles captures energy from visible light.
“In the absence of light, the copper-iron catalyst demonstrated approximately 300 times lower reactivity than copper-ruthenium catalysts, which is not surprising given that ruthenium is a better thermocatalyst for this reaction,” said Robatjazi, a Ph.D. alumnus from Halas’ research group who is now chief scientist at Houston-based Syzygy Plasmonics. “Under illumination, the copper-iron efficiencies and reactivities were comparable to and similar to those of copper-ruthenium.”
Syzygy has licensed Rice’s antenna-reactor technology, and the study included scaled-up tests of the catalyst in the company’s commercially available, LED-powered reactors. In laboratory tests at Rice, the copper-iron catalysts had been illuminated with lasers. The Syzygy tests showed the catalysts retained their efficiency under LED illumination and at a scale 500 times larger than lab setup.
“This is the first report in the scientific literature demonstrating that photocatalysis with LEDs can generate gram-scale quantities of hydrogen gas from ammonia,” Halas said. “This opens the door for precious metals to be completely replaced in plasmonic photocatalysis.”
“Plasmonic antenna-reactor photocatalysts are worthy of further investigation given their potential to significantly reduce chemical sector carbon emissions,” Carter added. “These outcomes are extremely motivating. They believe that other combinations of abundant metals could be used as low-cost catalysts for a variety of chemical reactions.”