On-chip nanophotonic devices are a type of device that can control light on a chip to achieve performance advantages over standard integrated photonics building blocks. These ultra-fast and low-power nanoscale optoelectronic devices are intended for use in high-performance computing, chemical and biological sensing technologies, energy-efficient lighting, environmental monitoring, and other applications. They are becoming an increasingly appealing building block in a variety of systems, owing to their unique characteristics of large evanescent field, compactness, and, most importantly, their ability to be configured according to the required application.
Researchers have created highly efficient on-chip frequency shifters capable of converting light at gigahertz frequencies. Using continuous and single-tone microwaves, the frequency shifters are easily controlled.
The ability to precise control and change photon properties such as polarization, position in space, and arrival time gave rise to a wide range of communication technologies that we use today, including the Internet. The next generation of photonic technologies, such as photonic quantum networks and computers, will necessitate even greater control over a photon’s properties.
One of the most difficult properties to change is a photon’s color, also known as its frequency because changing a photon’s frequency means changing its energy. Most frequency shifters today are either inefficient, losing a lot of light during the conversion process, or they can’t convert light in the gigahertz range, which contains the most important frequencies for communications, computing, and other applications.
Our frequency shifters could become a fundamental building block for high-speed, large-scale classical communication systems as well as emerging photonic quantum computers. We can achieve this magnitude of frequency shift with just a single 30-gigahertz microwave signal.
Marko Lonar
Harvard John A. Paulson School of Engineering and Applied Sciences (SEAS) researchers have developed highly efficient on-chip frequency shifters capable of converting light in the gigahertz frequency range. Using continuous and single-tone microwaves, the frequency shifters are easily controlled.
“Our frequency shifters could become a fundamental building block for high-speed, large-scale classical communication systems as well as emerging photonic quantum computers,” said Marko Lonar, senior author of the paper and the Tiantsai Lin Professor of Electrical Engineering.
The paper describes two types of on-chip frequency shifters: one that can convert one color to another with a few dozen gigahertz shifts, and another that can cascade multiple shifts with a shift of more than 100 gigahertz. Each device is based on Lonar’s and his lab’s lithium niobate platform.
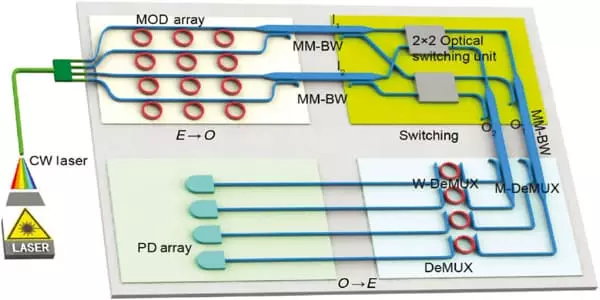
Although lithium niobate can efficiently convert electronic signals into optical signals, it has long been thought to be difficult to work with on small scales by many in the field. Lonar and his colleagues previously demonstrated a method for fabricating high-performance lithium niobate microstructures using standard plasma etching to physically sculpt microresonators in thin lithium niobate films.
Lonar and his colleagues etched coupled ring-resonators and waveguides on thin-film lithium niobate using the same technique. Two coupled resonators form a figure eight-like structure in the first device. Input light travels in a figure-eight pattern from the waveguide to the resonators, entering as one color and exiting as another. This device can provide frequency shifts of up to 28 gigahertz with approximately 90% efficiency. It can also be reconfigured as tunable frequency-domain beam splitters, splitting a beam of one frequency into two beams of another.
The second device employs three coupled resonators: a small ring resonator, a racetrack resonator, and a rectangular-shaped resonator. As light speeds around the racetrack resonator, it cascades into higher and higher frequencies, resulting in a 120 gigahertz shift.
“We can achieve this magnitude of frequency shift with just a single 30-gigahertz microwave signal,” said Yaowen Hu, a research assistant at SEAS and the paper’s first author. “This is an entirely new kind of photonic device. Previous attempts to shift frequencies by more than 100 gigahertz were difficult and expensive, necessitating an equally large microwave signal.”
“All of our previous developments in integrated lithium niobate photonics have made this work possible,” said Lonar. “The ability to process frequency-domain information in an efficient, compact, and scalable manner has the potential to significantly reduce the expense and resource requirements for large-scale photonic circuits, including quantum computing, telecommunications, radar, optical signal processing, and spectroscopy.”
The intellectual property associated with this project has been protected by Harvard’s Office of Technology Development, and commercialization opportunities are being pursued.