The blood-brain barrier, a protective cell layer that surrounds the brain, not only inhibits foreign bodies but also impedes medicine delivery, complicating the treatment of neurological illnesses. Scientists have developed a way for delivering mRNA into the brain using lipid nanoparticles (LNPs), which could advance treatments for Alzheimer’s disease and seizures.
Consider the brain to be an air traffic control tower, in charge of the body’s critical and complex ‘airport.’ This tower, which is critical for coordinating the constant flow of neurological signals, is guarded by a formidable layer that acts similarly to the airport’s security crew, meticulously screening everything and every one to ensure no unwelcome visitors disrupt the key workings inside.
However, this security, while vital, comes with a significant drawback: sometimes, a ‘mechanic’ — in the form of critical medication needed for treating neurological disorders — is needed inside the control tower to fix arising issues. But if the security is too stringent, denying even these essential agents entry, the very operations they’re meant to protect could be jeopardized.
Our model performed better at crossing the blood-brain barrier than others and helped us identify organ-specific particles that we later validated in future models. It’s an exciting proof of concept that will no doubt inform novel approaches to treating conditions like traumatic brain injury, stroke, and Alzheimer’s.
Michael Mitchell
Now, researchers led by Michael Mitchell of the University of Pennsylvania are breaking down this long-standing biological barrier, called as the blood-brain barrier, by creating a way similar to supplying this mechanic with a special keycard to overcome security. Their findings, published in the journal Nano Letters, describe a model that uses lipid nanoparticles (LNPs) to distribute mRNA, providing fresh hope for treating illnesses such as Alzheimer’s disease and seizures – similar to repairing the control tower’s flaws without jeopardizing its security.
“Our model performed better at crossing the blood-brain barrier than others and helped us identify organ-specific particles that we later validated in future models,” says Mitchell, associate professor of bioengineering at Penn’s School of Engineering and Applied Science, and senior author on the study. “It’s an exciting proof of concept that will no doubt inform novel approaches to treating conditions like traumatic brain injury, stroke, and Alzheimer’s.”
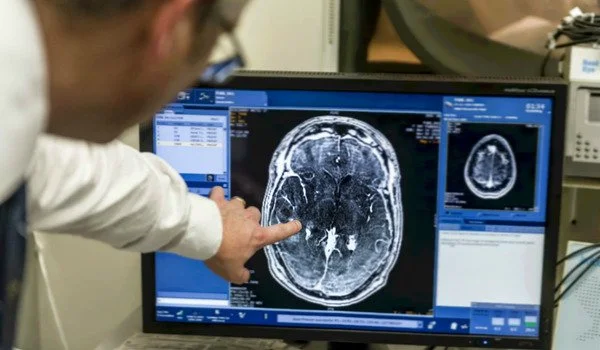
Search for the key
To develop the model, Emily Han, a Ph.D. candidate and NSF Graduate Research Fellow in the Mitchell Lab and first author of the paper, explains that it started with a search for the right in vitro screening platform, saying, “I was combing through the literature, most of the platforms I found were limited to a regular 96-well plate, a two-dimensional array that can’t represent both the upper and lower parts of the blood-brain barrier, which correspond to the blood and brain, respectively.”
Han then explored high-throughput transwell systems with both compartments but found they didn’t account for mRNA transfection of the cells, revealing a gap in the development process. This led her to create a platform capable of measuring mRNA transport from the blood compartment to the brain, as well as transfection of various brain cell types including endothelial cells and neurons.
“I spent months figuring out the optimal conditions for this new in vitro system, including which cell growth conditions and fluorescent reporters to use,” Han explains. “Once robust, we screened our library of LNPs and tested them on animal models. Seeing the brains express protein as a result of the mRNA we delivered was thrilling and confirmed we were on the right track.”
The team’s technology has the potential to greatly advance therapies for neurological illnesses. It is currently designed to examine a variety of LNPs containing brain-targeted peptides, antibodies, and different lipid compositions. However, it has the potential to deliver various therapeutic agents such as siRNA, DNA, proteins, or small molecule medicines directly to the brain following intravenous delivery.
Furthermore, this method is not confined to the blood-brain barrier, as it shows potential in developing treatments for pregnancy-related diseases by targeting the blood-placental barrier, as well as retinal diseases by targeting the blood-retinal barrier.
Next Steps
The team is excited to use this platform to screen new designs and assess their efficacy in various animal models. They are particularly interested in working with collaborators that have advanced animal models of neurological diseases.
“We’re collaborating with researchers at Penn to establish brain disease models,” Han said. “We’re looking into how these LNPs affect mice with a variety of brain diseases, from glioblastoma to traumatic brain traumas. We aim to make progress toward repairing the blood-brain barrier or targeting neurons that have been damaged after an accident.”