When you save a photograph to your smartphone, the information is put onto tiny transistors that are electrically turned on and off in a sequence of “bits” to represent and encode the image. Most modern transistors are composed of silicon, a material that scientists have been able to switch at ever-smaller sizes, allowing billions of bits, and therefore enormous libraries of pictures and other information, to be crammed into a single memory chip.
However, as the need for data and the means to store it grows, scientists are looking for materials other than silicon that can drive memory devices to better densities, speeds, and security.
Now, MIT physicists have provided early evidence that data may be stored in antiferromagnet bits that are quicker, denser, and more secure.
The lesser-known relatives of ferromagnets, or typical magnetic materials, are antiferromagnetic, or AFM, materials. Whereas electrons in ferromagnets spin in lockstep, allowing a compass needle to point north by collectively following the Earth’s magnetic field, electrons in antiferromagnets prefer the opposite spin, resulting in “antialignment,” which effectively quenches magnetization even at the smallest scales.
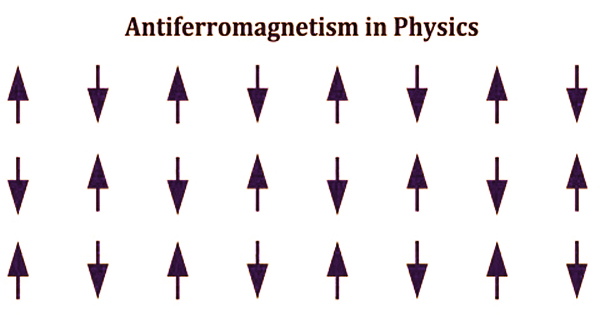
Antiferromagnets are resistant to external magnetic fields due to their lack of net magnetism. Antiferromagnetic bits, if turned into memory devices, may safeguard any encoded data from being magnetically wiped. They could also be manufactured into smaller transistors with a higher number of transistors per chip than conventional silicon.
The MIT team has discovered that by doping additional electrons into an antiferromagnetic material, they can controllably turn the material’s collective antialigned arrangement on and off. They discovered that this magnetic transition is reversible and sharp, akin to altering the state of a transistor from 0 to 1. The findings, which were published today in Physical Review Letters, show that antiferromagnets might be used as a digital switch in the future..
“An AFM memory could enable scaling up the data storage capacity of current devices same volume, but more data,” says the study’s lead author Riccardo Comin, assistant professor of physics at MIT.
Jiarui Li, lead author and graduate student at MIT, as well as Zhihai Zhu, Grace Zhang, and Da Zhou; Roberg Green of the University of Saskatchewan; Zhen Zhang, Yifei Sun, and Shriram Ramanathan of Purdue University; Ronny Sutarto and Feizhou He of Canadian Light Source; and Jerzy Sadowski of Brookhaven National Laboratory are among Comin’s co-authors.
Magnetic memory –
Some researchers are turning at MRAM, or magnetoresistive RAM, to enhance data storage. MRAM is a sort of memory system that stores data as bits made from ordinary magnetic materials. An MRAM device would be designed with billions of magnetic bits in theory. The direction of a local magnetic domain within the device is flipped to encode data, much as how a transistor is switched from 0 to 1.
MRAM systems might possibly read and write data quicker and use less power than silicon-based devices. They may, however, be susceptible to external magnetic fields.
“The system as a whole follows a magnetic field like a sunflower follows the sun, which is why, if you take a magnetic data storage device and put it in a moderate magnetic field, information is completely erased,” Comin says.
Antiferromagnets, on the other hand, are unaffected by external fields and so might be a safer alternative to MRAM systems. The ability to turn antiferromagnetism on and off is a crucial step toward encodable AFM bits. Researchers have devised a number of methods for accomplishing this, the most common of which involves switching a material from its ordered antialignment to a random disorder of spins using electric current.
“With these approaches, switching is very fast,” says Li. “The disadvantage is that every time you need a current to read or write, it takes a lot of energy. Running currents create a lot of energy and heat when objects grow really tiny.”
Doped disorder –
Comin and his colleagues wondered if they might improve the efficiency of antiferromagnetic switching. They use neodymium nickelate, an antiferromagnetic oxide produced at the Ramanathan lab, in their new research. Nanodomains made up of nickel atoms with opposing spins to their neighbors are kept together by oxygen and neodymium atoms in this material. The fractal characteristics of the material have already been mapped by the researchers.
Since then, the researchers have been attempting to modify the material’s antiferromagnetism via doping, a technique that involves purposefully introducing impurities into a material in order to change its electrical characteristics. The researchers didped neodymium nickel oxide by removing the oxygen atoms from the substance.
When an oxygen atom is removed, two electrons are left behind, which are redistributed among the remaining nickel and oxygen atoms. The researchers questioned if removing a large number of oxygen atoms would cause a domino cascade of disorder, thereby turning off the material’s ordered antialignment.
They put 100-nanometer-thin films of neodymium nickel oxide in an oxygen-depleted room to test their idea, then heated the samples to 400 degrees Celsius to stimulate oxygen to escape from the films and into the chamber’s environment.
They used sophisticated magnetic X-ray crystallography techniques to examine the films as they eliminated additional oxygen to see if the material’s magnetic structure was intact, suggesting that its atomic spins stayed in their ordered antialignment and therefore preserved antiferromagnetism. If their data revealed that the material’s antiferromagnetism had turned off owing to adequate doping, it would constitute proof that the material’s antiferromagnetism had switched off.
The researchers were able to turn off the material’s antiferromagnetism at a crucial doping threshold through their tests. They may potentially reintroduce antiferromagnetism into the material by reintroducing oxygen.
Scientists may utilize more realistic techniques to dope comparable materials now that the team has demonstrated that doping efficiently switches AFM on and off. Voltage-activated “gates,” for example, are used to switch silicon-based transistors, where a tiny voltage is given to a bit to change its electrical conductivity. Antiferromagnetic bits may also be switched using appropriate voltage gates, according to Comin, which would need less energy than previous antiferromagnetic switching approaches.
“This could present an opportunity to develop a magnetic memory storage device that works similarly to silicon-based chips, with the added benefit that you can store information in AFM domains that are very robust and can be packed at high densities,” Comin says. “That’s key to addressing the challenges of a data-driven world.”