The National Institute of Standards and Technology (NIST) has revived and refined a once-reliable technology for identifying and counting faults in transistors, which are the building blocks of contemporary electronic gadgets such as smartphones and computers. Over the last decade, transistor components in high-performance computer circuits have become so small that the traditional process known as charge pumping can no longer identify errors correctly.
The new and upgraded NIST approach is sensitive enough for the most recent, tiny technology, and it can provide an accurate assessment of faults that might otherwise impede transistor performance and limit the reliability of the chips in which they live.
The new, improved charge pumping technology may detect single flaws as small as the diameter of a hydrogen atom (one-tenth of a billionth of a meter) and pinpoint their location in the transistor. Researchers could also utilize the new capability to detect and modify a feature of each electron known as quantum spin. The ability to manipulate individual spins has applications in both basic research and quantum engineering and computation.
Transistors function as electrical switches. In the on state, which represents the “1” of binary digital information, a specific amount of current flows from one side of a semiconductor to the other. Current stops flowing when the switch is turned off, which represents the “0” of binary logic.
We’ve given charge pumping a new lease on life. The modulated-frequency approach is now helpful for looking at single interface faults, giving engineers control of single electron charges in a highly sensitive measuring system.
James Ashton
Defects in a transistor can disrupt the dependable flow of current and drastically reduce transistor performance. These flaws could be the result of broken chemical bonds in the transistor material. Or they could be atomic imperfections in the substance that trap electrons. Scientists have evolved a number of methods for categorizing faults and mitigating their impact, each adapted to the structure of the transistor under consideration.
A metal electrode called the gate lies above a thin insulating layer of silicon dioxide in the classic configuration known as the metal oxide semiconductor field-effect transistor (MOSFET). The interface area is located beneath the insulating layer and separates the insulating layer from the main body of the semiconductor. In a typical transistor, current flows via a narrow channel that is only one billionth of a meter thick and runs from the source on one side of the gate to a “drain” on the other. The gate regulates the amount of electricity flowing through the channel.
Charge pumping is a two-step procedure in which the examiner alternately pulses the gate with a positive and a negative test voltage. (During this testing mode, the transistor does not function as an on/off switch.) The alternating voltage pulses are applied at a single, fixed frequency in classical charge pumping.
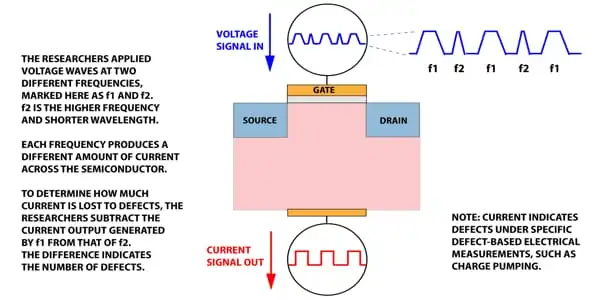
The positive voltage attracts or pumps negatively charged electrons to the boundary or interface between the gate’s insulating layer and the body of the transistor in the first step of the test. Some of the pumped electrons become trapped in interface defects, but there are a lot of electrons left over. A negative voltage is provided in the second stage to clear the contact of surplus electrons, leaving just the trapped ones behind. The negative voltage also attracts positive charge carriers, referred to as “holes,” to the region, where they join with electrons trapped in the defects. The current generated by this action is proportional to the number of faults. The higher the output current, the more flaws there are.
In the recent past, the current was a reliable gauge of faults. However, the insulating oxide layer in modern transistors is now so thin — about 10 to 20 hydrogen atoms broad — that a quantum mechanics effect enters the picture, confusing observations using the old charge-pumping method.
According to quantum theory, electrons and other subatomic particles can never be totally confined; there is always the possibility that they will escape or “tunnel” out of an enclosure or boundary layer. The thinner the material, the more likely it is that electrons may escape, resulting in a tunneling current. As transistor size dropped, the tunneling current flowing through the insulating oxide layer made it practically difficult to identify flaws with conventional charge pumping. Scientists all but abandoned the technique.
NIST researchers James Ashton, Mark Anders, and Jason Ryan have discovered a way to improve the approach such that it not only works for ultrathin transistor components but is also more sensitive, allowing scientists to record signals from a single flaw. The solution emerged when the scientists realized something important: the current produced by quantum tunneling remains nearly constant regardless of the frequency at which charge pumping pulses the positive and negative voltages.
Armed with this knowledge, the team changed the charge pumping technique by applying the system’s positive and negative voltages at two different frequencies instead of the one frequency employed in the old method. The researchers obtained two different output currents by applying the voltages at two different frequencies. The constant signal from the quantum tunneling current was removed by subtracting one output current from the other. The researchers were able to discover faults in transistors with ultrasmall features after removing the perplexing tunneling current. The researchers published their work on the frequency-modulated charge pumping approach in the journal Applied Physics Letters.
“We’ve given charge pumping a new lease on life,” Ashton added. “The modulated-frequency approach is now helpful for looking at single interface faults, giving engineers control of single electron charges in a highly sensitive measuring system,” he noted. Because only one electron is involved, the output current is equal to multiples of the electron’s charge, a fundamental physics constant determined by NIST and other institutes.
Because the approach can detect single electrons, it might be used as a sensitive probe of an electron’s quantum spin. Scientists investigating how electron spin can store and convey information in a future computer may find a beneficial guide in modulated-frequency charge pumping. It may also prove useful in quantum metrology, as a potential new way of determining a quantum standard of electrical current.