Lab-grown organs have long been regarded as the ‘holy grail’ of organ engineering, but recent study has moved that objective a step closer to reality by utilizing a new 3D-printing technology known as co-SWIFT. co-SWIFT prints branching networks of double-layered arteries injected with smooth muscle cells and endothelial cells into real human heart tissue, and it can even recreate patient-specific vascular patterns, implying that it could one day be employed in personalized therapy.
Growing functional human organs outside the body has long been regarded as the “holy grail” of organ transplantation treatment, yet it remains elusive. New research from Harvard’s Wyss Institute for Biologically Inspired Engineering and the John A. Paulson School of Engineering and Applied Science (SEAS) puts that quest one step closer to being realized.
A team of scientists devised a new technology for 3D printing vascular networks made up of interconnected blood arteries with a distinct “shell” of smooth muscle cells and endothelial cells surrounding a hollow “core” through which fluid can flow, placed inside human cardiac tissue. This vascular architecture closely resembles that of naturally existing blood arteries and represents a big step toward the ability to construct implantable human organs. The achievement was published in Advanced Materials.
We were able to successfully 3D-print a model of the vasculature of the left coronary artery based on data from a real patient, which demonstrates the potential utility of co-SWIFT for creating patient-specific, vascularized human organs.
Jennifer Lewis
“In prior work, we developed a new 3D bioprinting method, known as “sacrificial writing in functional tissue” (SWIFT), for patterning hollow channels within a living cellular matrix. Here, building on this method, we introduce coaxial SWIFT (co-SWIFT) that recapitulates the multilayer architecture found in native blood vessels, making it easier to form an interconnected endothelium and more robust to withstand the internal pressure of blood flow,” said first author Paul Stankey, a graduate student at SEAS in the lab of co-senior author and Wyss Core Faculty member Jennifer Lewis, Sc.D.
The team’s main innovation was a novel core-shell nozzle with two independently controlled fluid channels for the “inks” that make up the printed vessels: a collagen-based shell ink and a gelatin-based core ink. The inside core chamber of the nozzle extends slightly beyond the shell chamber, allowing the nozzle to entirely puncture a previously printed channel, forming interconnected branching networks for adequate oxygenation of human tissues and organs via perfusion. The size of the vessels can be changed during printing by adjusting the printing speed or ink flow rates.
To confirm the new co-SWIFT method worked, the team first printed their multilayer vessels into a transparent granular hydrogel matrix. Next, they printed vessels into a recently created matrix called uPOROS composed of a porous collagen-based material that replicates the dense, fibrous structure of living muscle tissue. They were able to successfully print branching vascular networks in both of these cell-free matrices. After these biomimetic vessels were printed, the matrix was heated, which caused collagen in the matrix and shell ink to crosslink, and the sacrificial gelatin core ink to melt, enabling its easy removal and resulting in an open, perfusable vasculature.
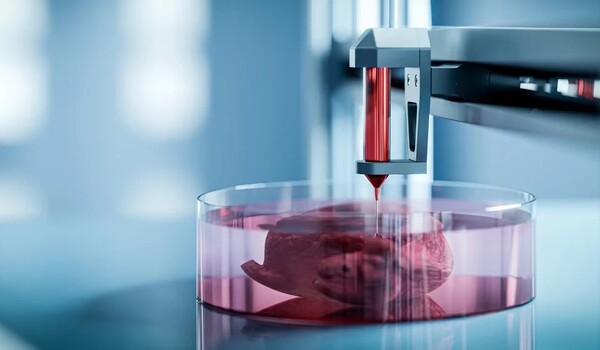
Moving into even more biologically relevant materials, the team repeated the printing process using a shell ink that was infused with smooth muscle cells (SMCs), which comprise the outer layer of human blood vessels. After melting out the gelatin core ink, they then perfused endothelial cells (ECs), which form the inner layer of human blood vessels, into their vasculature. After seven days of perfusion, both the SMCs and the ECs were alive and functioning as vessel walls – there was a three-fold decrease in the permeability of the vessels compared to those without ECs.
They were finally ready to put their technology to the test in living human tissue. They created hundreds of thousands of cardiac organ building blocks (OBBs), which are tiny spheres of beating human heart cells packed into a dense cellular matrix. Then, via co-SWIFT, they printed a biomimetic vascular network into the cardiac tissue. Finally, they removed the sacrificial core ink and seeded the inner surface of their SMC-laden vessels with ECs by perfusion before assessing their performance.
Not only did these printed biomimetic vessels display the characteristic double-layer structure of human blood vessels, but after five days of perfusion with a blood-mimicking fluid, the cardiac OBBs started to beat synchronously – indicative of healthy and functional heart tissue. The tissues also responded to common cardiac drugs — isoproterenol caused them to beat faster, and blebbistatin stopped them from beating. The team even 3D-printed a model of the branching vasculature of a real patient’s left coronary artery into OBBs, demonstrating its potential for personalized medicine.
“We were able to successfully 3D-print a model of the vasculature of the left coronary artery based on data from a real patient, which demonstrates the potential utility of co-SWIFT for creating patient-specific, vascularized human organs,” said Lewis, the Hansjörg Wyss Professor of Biologically Inspired Engineering at SEAS.
Lewis’ team intends to produce self-assembled capillary networks and combine them with their 3D-printed blood vessel networks in the future to better mimic the shape of human blood vessels on the microscale and improve the function of lab-grown tissues.
“To say that engineering functional living human tissues in the lab is difficult is an understatement. I’m proud of the determination and creativity this team showed in proving that they could indeed build better blood vessels within living, beating human cardiac tissues. I look forward to their continued success on their quest to one day implant lab-grown tissue into patients,” said Wyss Founding Director Donald Ingber, M.D., Ph.D. Ingber is also the Judah Folkman Professor of Vascular Biology at HMS and Boston Children’s Hospital and Hansjörg Wyss Professor of Biologically Inspired Engineering at SEAS.