The precise origin of Earth’s water is still being researched and debated. However, one popular theory holds that comets and asteroids brought water to Earth during the planet’s early formation.
The early Earth, according to this theory, was a hot and dry place with no water on its surface. Ice comets and asteroids collided with the planet as it cooled, delivering water and other volatile compounds that eventually accumulated in the planet’s atmosphere and oceans.
According to new research from Carnegie Science’s Anat Shahar and UCLA’s Edward Young and Hilke Schlichting, our planet’s water could have originated from interactions between the hydrogen-rich atmospheres and magma oceans of the planetary embryos that comprised Earth’s formative years. Their findings, which may explain the origins of Earth’s signature features, have been published in the journal Nature.
For decades, researchers’ understanding of planet formation was primarily based on our own Solar System. Although there are some active debates about the formation of gas giants such as Jupiter and Saturn, it is widely accepted that Earth and the other rocky planets formed from the disk of dust and gas that surrounded our Sun in its early years.
As larger and larger objects collided, the baby planetesimals that eventually formed Earth grew larger and hotter, melting into a vast magma ocean due to the heat of collisions and radioactive elements. As the planet cooled, the densest material sank inward, separating Earth into three distinct layers: the metallic core, the rocky, silicate mantle, and the crust. However, the recent explosion in exoplanet research has informed a new approach to modeling the Earth’s embryonic state.
This is just one possible explanation for our planet’s evolution, but one that would establish an important link between Earth’s formation history and the most common exoplanets that have been discovered orbiting distant stars, which are called Super-Earths and sub-Neptunes.
Anat Shahar
“Exoplanet discoveries have given us a much better appreciation of how common it is for newly formed planets to be surrounded by atmospheres rich in molecular hydrogen, H2, during their first several million years of growth,” Shahar explained. “Eventually these hydrogen envelopes dissipate, but they leave their fingerprints on the young planet’s composition.”
Using this data, the scientists created new models of Earth’s formation and evolution to see if our home planet’s distinct chemical characteristics could be replicated. Using a newly developed model, the Carnegie and UCLA researchers demonstrated that interactions between the magma ocean and a molecular hydrogen proto-atmosphere could have given rise to some of Earth’s signature features, such as its abundance of water and overall oxidized state, early in Earth’s existence.
The researchers used mathematical modeling to investigate the exchange of materials between molecular hydrogen atmospheres and magma oceans, examining 25 different compounds and 18 different types of reactions – complex enough to yield valuable information about Earth’s possible formative history, but simple enough to fully interpret.
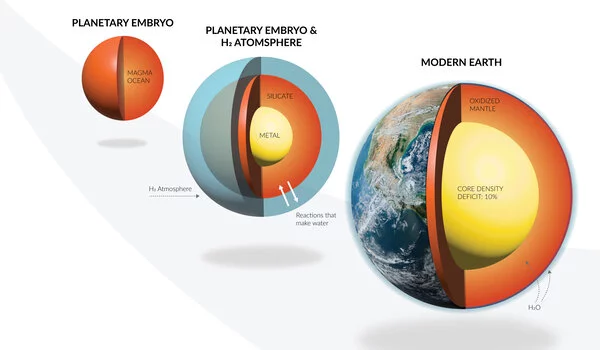
Interactions between the magma ocean and the atmosphere in their simulated baby Earth resulted in the movement of large masses of hydrogen into the metallic core, mantle oxidation, and the production of large amounts of water.
Even if all of the rocky material that collided to form the growing planet was completely dry, the researchers discovered that interactions between the molecular hydrogen atmosphere and the magma ocean would generate copious amounts of water. Other water sources, they argue, are possible but not required to explain Earth’s current state.
“This is just one possible explanation for our planet’s evolution, but one that would establish an important link between Earth’s formation history and the most common exoplanets that have been discovered orbiting distant stars, which are called Super-Earths and sub-Neptunes,” Shahar concluded.
This project was part of Shahar’s interdisciplinary, multi-institution AEThER project, which seeks to reveal the chemical makeup of the Milky Way galaxy’s most common planets – Super-Earths and sub-Neptunes – as well as to develop a framework for detecting life’s signatures on distant worlds. The Alfred P. Sloan Foundation funded this effort to learn more about how the formation and evolution of these planets shape their atmospheres. This, in turn, could allow scientists to distinguish true biosignatures, which can only be produced by the presence of life, from non-biological atmospheric molecules.
“Increasingly powerful telescopes are enabling astronomers to understand the compositions of exoplanet atmospheres in never-before-seen detail,” Shahar said. “We hope that AETHER’s work will inform their observations with experimental and modeling data, leading to a foolproof method for detecting signs of life on other worlds.”