An international team of laser physicists from LMU and the Max Planck Institute of Quantum Optics has achieved unprecedented control over light pulses in the mid-infrared wavelength range.
Ultrashort infrared light pulses are essential for a wide range of technological applications. The oscillating infrared light field can excite molecules in a sample to vibrate at specific frequencies or drive ultrafast electric currents in semiconductors. Anyone who wants to use the oscillating waveform of ultrashort light pulses to drive cutting-edge electro-optical processes, for example, must first figure out how to control the waveform itself.
In different wavelength ranges, such as the UV-visible and near-infrared, the generation of ultrashort pulses with adjustable waveforms has been demonstrated. Physicists from the LMU’s attoworld team, the Max Planck Institute of Quantum Optics (MPQ), and the Hungarian Center for Molecular Fingerprinting (CMF) have created ultrashort mid-infrared pulses and precisely controlled their electric-field waveforms. With this infrared waveform manipulator in hand, new optical control possibilities for biomedical applications and quantum electronics become possible.
With our laser technology, we have significantly expanded the controllable wavelength range in the infrared. The additional wavelengths allow us to analyze a mixture of molecules even more precisely.
Nathalie Nagl
A stabilized laser system that generates light pulses with a precisely defined waveform at near-infrared wavelengths serves as the foundation for the new mid-infrared source. The pulses are only a few femtoseconds long because they are made up of only one oscillation of the light wave. When these pulses are sent into a suitable nonlinear crystal, complex frequency-mixing processes can be used to induce the generation of long-wavelength infrared pulses.
The team was able to create light pulses with an unusually broad spectral coverage of more than three optical octaves, ranging from 1 to 12 micrometres. The researchers were not only able to understand and simulate the underlying physics of the mixing processes, but also developed a new approach to precisely control the oscillations of the generated mid-infrared light via the tuning of the laser input parameters.
Infrared light is a door opener for a variety of technological applications. It creates the prerequisites for specifically stimulating molecules to vibrate and for generating electrical signals in semiconductors. If you want to technically use the oscillation of electric fields from ultra-short light pulses, you first have to find out how to best control them. This works well in many wavelength ranges, but until now controlling and controlling light in the mid-infrared wavelength spectrum has been a challenge.
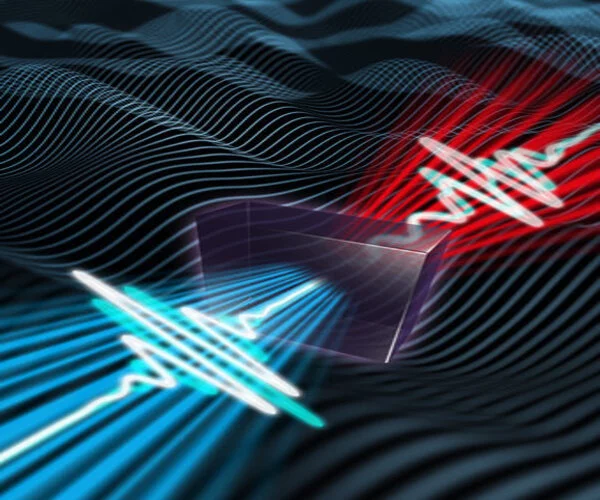
The resulting adjustable waveforms, for example, can selectively trigger specific electronic processes in solids, potentially allowing for much faster electronic signal processing speeds in the future. “On this basis, one could imagine the development of light-controlled electronics,” says Philipp Steinleitner, one of the study’s three lead authors. “By operating opto-electronic devices at the frequencies of the generated light, you could speed up today’s electronics by at least a factor of 1000.”
Attoworld physicists are particularly interested in the use of new light technology for molecule spectroscopy. When mid-infrared light passes through a sample liquid, such as human blood, the molecules in the sample begin to oscillate and emit characteristic light waves. Detecting the molecular response yields a unique fingerprint that is dependent on the exact composition of the sample.
“With our laser technology, we have significantly expanded the controllable wavelength range in the infrared,” says Nathalie Nagl, the study’s first author. “The additional wavelengths allow us to analyze a mixture of molecules even more precisely,” she continues.
Colleagues from the Broadband Infrared Diagnostics (BIRD) team led by Mihaela Zigman and the CMF Research team led by Alexander Weigel in the attoworld group are particularly interested in measuring the precise infrared molecular fingerprints of human blood samples. The goal is to identify distinctive signatures that can be used to diagnose diseases such as cancer. A growing tumor, for example, causes minute and highly complex changes in the molecular composition of the blood. By measuring the infrared fingerprint of a simple drop of human blood, the goal is to detect these changes and enable early disease diagnosis.
“Our laser technology will enable our colleagues to detect previously undetectable changes in specific biomolecules such as proteins or lipids in the future. As a result, future medical diagnostics using infrared laser technology will be more reliable” Maciej Kowalczyk, the study’s first author, agrees.