Color centers are crystal lattice defects that can capture one or more additional electrons. These electrons’ spin is extremely sensitive to external electric and magnetic fields, as well as sound. Sound has been used to selectively manipulate electron spins in both their ground and excited states, according to researchers. Their approach paves the way for previously unattainable methods of processing quantum information.
The captured electrons typically absorb light in the visible spectrum, causing a transparent material, such as diamond, to become colored in the presence of such centers. “Color centers frequently have magnetic properties, making them promising systems for applications in quantum technologies such as quantum memories (qubits) or quantum sensors. The challenge here is to devise efficient methods for controlling electrons’ magnetic quantum properties, or, in this case, their spin states “Dr. Georgy Astakhov of the Institute of Ion Beam Physics and Materials Research at HZDR explains.
You could think of this control as tuning a guitar with a regular electronic tuner. Only that in our experiment it is a bit more complicated: a magnetic field tunes the resonant frequencies of the electron spin to the frequency of the acoustic wave, while a laser induces transitions between the ground and excited states of the color center.
Dr. Alexander Poshakinskiy
Dr. Alberto Hernández-Mnguez of the Paul-Drude-Institut elaborates on the subject: “This is typically accomplished through the use of electromagnetic fields, but mechanical vibrations such as surface acoustic waves can also be used. These are sound waves that are confined to the surface of a solid and look like water waves on a lake. In modern electronic devices such as mobile phones, tablets, and laptops, they are commonly integrated into microchips as radio frequency filters, oscillators, and transformers.”
Tuning the spin to the sound of a surface
The researchers demonstrate the use of surface acoustic waves for on-chip control of electron spins in silicon carbide, a semiconductor that will replace silicon in many applications requiring high-power electronics, such as electrical vehicles, in their paper. “You could think of this control as tuning a guitar with a regular electronic tuner,” says Dr. Alexander Poshakinskiy of the Ioffe Physical-Technical Institute in St. Petersburg, adding, “only that in our experiment it is a bit more complicated: a magnetic field tunes the resonant frequencies of the electron spin to the frequency of the acoustic wave, while a laser induces transitions between the ground and excited states of the color center.”
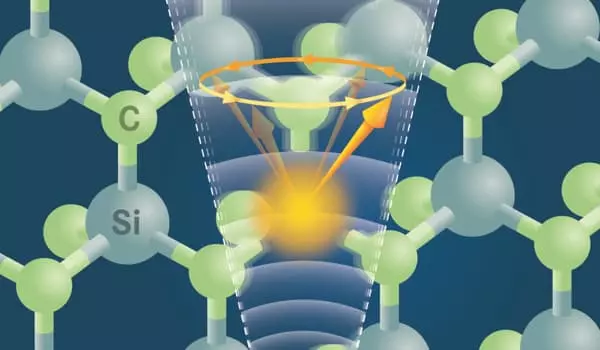
These optical transitions are critical because they allow for the optical detection of the spin state by registering the light quanta emitted when the electron returns to the ground state. The scientists achieve simultaneous control of the electron spin by the acoustic wave in both its ground and excited states thanks to a massive interaction between the periodic vibrations of the crystal lattice and the electrons trapped in the color centers.
At this point, Hernández-Mnguez introduces a new physical process: precession “Precession was first observed as a change in the orientation of the rotational axis while attempting to tilt a spinning top as a child. An electronic spin can also be thought of as a tiny spinning top, with precession axes influenced by an acoustic wave that changes orientation every time the color center switches between ground and excited states. Because the amount of time the color center spends in the excited state is random, the large difference in the alignment of the precession axes in the ground and excited states changes the orientation of the electron spin in an uncontrollable way.”
After several jumps, the quantum information stored in the electronic spin is lost as a result of this change. The researchers demonstrate a way to avoid this in their work: by appropriately tuning the resonant frequencies of the color center, the precession axes of the spin in the ground and excited states become collinear: the spins maintain their precession orientation along a well-defined direction even when they jump between the ground and excited states.
The quantum information stored in the electron spin becomes decoupled from the laser’s jumps between the ground and excited states under this specific condition. This acoustic manipulation technique opens up new possibilities for processing quantum information in quantum devices with dimensions similar to current microchips. This should have a significant impact on fabrication costs and, as a result, the general public’s access to quantum technologies.