Metallic nanostructures’ ability to confine light at the sub-wavelength scale opens up new avenues and opportunities in the field of nanotechnology. Taking advantage of this distinct advantage, nano-optical trapping techniques have been developed to address new challenges in a variety of fields ranging from biology to quantum optics.
Plasmonic nanostructures have recently received a lot of attention because of their ability to overcome the diffraction limit of dielectric configurations and, as a result, have been used to manipulate nanosized particles. They have the ability to provide strong, sub-wavelength energy confinement with a high optical gradient at the interface between the plasmonic and dielectric layers, making them suitable for novel applications in optical communications, optical imaging, energy harvesting, nanoelectronics, sensing, and optical trapping.
Physicists from the University of Southampton and the Swiss Federal Institute of Technology have achieved a new level of light-matter coupling at the nanoscale. The international study, which was published in Nature Photonics, combined theoretical and experimental findings to identify a fundamental limitation in our ability to confine and exploit light.
The collaboration centered on photonic nano-antennas made in ever-smaller sizes on top of a two-dimensional electron gas. The setup is commonly used in laboratories around the world to investigate the effect of intense electromagnetic coupling, taking advantage of the antennas’ ability to trap and focus light near electrons.
Physicists have reached a new threshold of light-matter coupling at the nanoscale. The collaboration focussed on photonic nano-antennas fabricated in ever reducing sizes on the top of a two-dimensional electron gas.
We have seen rapid progress in the development of organic photovoltaic devices over the last decade (OPVs). At the moment, the highest level of efficiency has surpassed 10%, indicating that OPVs have a high potential to compete with other thin-film solar technologies.
“The fabrication of photonic resonators capable of focusing light in extremely small volumes is proving a key technology that is currently enabling advances in fields as diverse as material science, optoelectronics, chemistry, quantum technologies, and many others,” says Professor Simone De Liberato, Director of the Quantum Theory and Technology group at the University of Southampton.
“Focused light, in particular, can be made to interact extremely strongly with matter, rendering electromagnetism non-perturbative. Light can then be used to modify the properties of the materials with which it interacts, transforming it into a powerful tool for material science. Light can be successfully woven into new materials.”
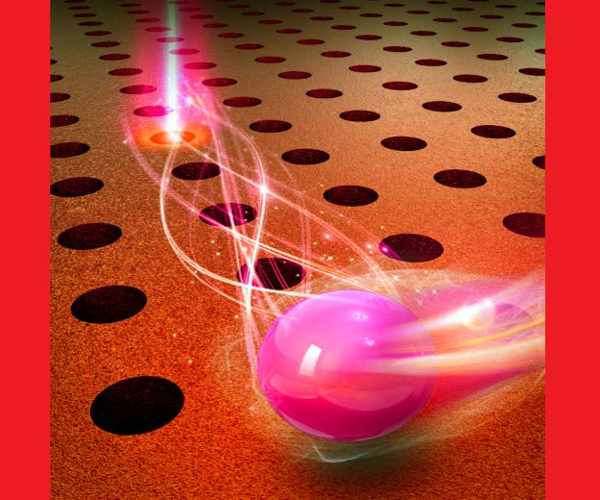
When the experiment began to excite propagating plasmons, scientists discovered that light could no longer be confined in the system below a critical dimension of the order of 250nm in the sample under study. This caused electron waves to move away from the resonator, spilling the photon’s energy.
The gain of nanoscale photoconductors is inversely proportional to the surface trap states. The density of surface trap states in the semiconductor bandgap must be calculated in order to engineer the performance of nanoscale photoconductors. Traditional capacitive techniques for measuring surface trap states are unsuitable for nanoscale devices.
Experiments conducted at ETH Zürich by Professors Jérôme Faist and Giacomo Scalari yielded results that could not be explained using the current understanding of light-matter coupling. The physicists approached Southampton’s School of Physics and Astronomy, where researchers led theoretical analysis and developed a novel theory capable of reproducing the results quantitatively.
Professor De Liberato believes that future experiments could push the newfound limits even further, enabling dramatic technological advances based on ultra-confined electromagnetic fields.
“It’s been said that proofs of impossibility are just proofs of a lack of imagination,” he says. “This isn’t the first time a ‘fundamental limit’ on how tightly we can focus light has been discovered.” The most famous is the Abbe diffraction limit, named after German physicist Ernst Abbe in the nineteenth century, which states that light cannot be confined in a volume smaller than a cubic wavelength.
“Nanophotonics is a very active and successful field of research that is investigating various methods to overcome the Abbe limit. I believe the next step will be to employ some ingenuity and look for novel ways to confine light, thereby avoiding both the Abbe limit and the one we have just discovered.”
Plasmonic nanotweezers with metallic nanoantennas are a powerful tool for trapping nanoscale particles, but the strong heating effect caused by light absorption limits their use.