Super-resolution fluorescence microscopy (SRFM) is a technique that allows scientists to surpass the diffraction limit of light and obtain images with higher spatial resolution than traditional microscopy. One major advance in SRFM has been the development of a technique called stimulated emission depletion (STED) microscopy.
Scientists at the Max Planck Institute for Medical Research in Heidelberg, led by Nobel Laureate Stefan Hell, have developed a super-resolution microscope with a spatio-temporal precision of one nanometer per millisecond. The stepping motion of the motor protein kinesin-1 as it walks along microtubules while consuming ATP was observed in unprecedented detail using an improved version of their recently introduced MINFLUX super-resolution microscopy. The study demonstrates the power of MINFLUX as a game-changing new tool for observing nanometer-sized conformational changes in proteins.
Understanding the inner workings of a cell necessitates an understanding of the biochemistry of individual proteins. The main challenge here is measuring minute changes in their position and shape. In this emerging field, fluorescence microscopy, particularly super-resolution microscopy (i.e. nanoscopy), has become indispensable. The recently introduced fluorescence nanoscopy system, MINFLUX, has already achieved spatial resolutions of one to a few nanometers: the size of small organic molecules. However, progressing our understanding of molecular cell physiology necessitates observations with even higher spatiotemporal resolution.
One challenge is to build a MINFLUX microscope that works close to the theoretical limit and is noise-free. Creating probes that do not interfere with protein function while still revealing the biological mechanism is another.
Otto Wolff
MINFLUX was first presented by Stefan Hell’s group in 2016 and was used to track fluorescently labeled proteins in cells. However, these movements were random, and the tracking was accurate to tens of nanometers. Their research is the first to apply MINFLUX’s resolving power to protein conformational changes, specifically the motor protein kinesin-1. To accomplish this, Max Planck Institute for Medical Research researchers created a new MINFLUX version for tracking single fluorescent molecules.
All established methods for measuring protein dynamics have significant limitations, making it difficult to address the critical (sub)nanometer / (sub)millisecond range. Some provide high spatial resolution, down to a few nanometers, but are incapable of tracking changes quickly enough. Others have a high temporal resolution but necessitate labeling with beads that are 2 to 3 orders of magnitude larger than the protein under investigation. Because a bead of this size is likely to impair protein function, studies involving beads raise questions.
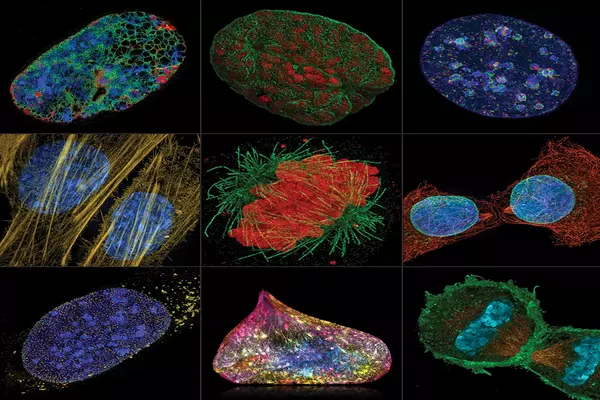
Fluorescence from a single molecule
MINFLUX, on the other hand, requires only a standard 1-nm fluorescence molecule as a label attached to the protein, allowing it to provide both the resolution and minimal invasiveness required for studying native protein dynamics. “One challenge is to build a MINFLUX microscope that works close to the theoretical limit and is noise-free,” says Otto Wolff, a PhD student in the group. “Creating probes that do not interfere with protein function while still revealing the biological mechanism is another,” his colleague Lukas Scheiderer adds.
The researchers’ new MINFLUX microscope can record protein movements with spatiotemporal precision of up to 1.7 nanometers per millisecond. It only takes about 20 photons emitted by the fluorescent molecule to detect it. “I believe we are starting a new chapter in the study of the dynamics of individual proteins and how they change shape during their function,” Stefan Hell says. “MINFLUX’s combination of high spatial and temporal resolution will enable researchers to study biomolecules like never before.”
Resolving the stepping motion of kinesin-1 with ATP under physiological conditions
Kinesin-1 is a key player in transporting cargo throughout our cells, and mutations of the protein are at the heart of several diseases. Kinesin-1 actually ‘walks’ along filaments (the microtubules) that span our cells like a network of streets. One can imagine the motion as literally ‘stepping’, since the protein has two ‘heads’ that alternately change their location on the microtubule. This movement occurs usually along one of the 13 protofilaments forming the microtubule, and is fueled by splitting of the cell’s principal energy supplier ATP (adenosine triphosphate).
The scientists recorded the regular 16 nm steps of individual heads as well as 8 nm substeps with nanometer/millisecond spatiotemporal resolution, using only a single fluorophore to label the kinesin-1. Their findings demonstrated that ATP is taken up when only one head is bound to a microtubule, but ATP hydrolysis occurs when both heads are bound. It also revealed that the stepping is caused by a rotation of the protein’stalk,’ which is the part of the kinesin molecule that holds the cargo. MINFLUX’s spatiotemporal resolution also revealed a head rotation in the initial phase of each step. Significantly, these findings were made using physiological concentrations of ATP, which had previously been impossible to achieve with tiny fluorescence labels.
Future potential in exploring protein dynamics
“I’m looking forward to seeing where MINFLUX takes us. It broadens our understanding of how proteins function. This can help us understand the mechanisms underlying many diseases and, eventually, contribute to the development of treatments “Jessica Matthias, a postdoctoral scientist in Hell’s group who is now investigating the applications of MINFLUX to a variety of biological questions, concurs.