For years, researchers have tried numerous methods to coax quantum bits or qubits, the basic building blocks of quantum computers, to remain in their quantum state for ever-longer periods of time, a critical step in developing devices such as quantum sensors, gyroscopes, and memories.
A group of MIT physicists has taken a crucial step ahead in that search, and they did so by borrowing an idea from an odd source: noise-canceling headphones.
The team described a method to achieve a 20-fold increase in the coherence times for nuclear-spin qubits, led by Ju Li, the Battelle Energy Alliance Professor in Nuclear Engineering and professor of materials science and engineering, and Paola Cappellaro, the Ford Professor of Engineering in the Department of Nuclear Science and Engineering and Research Laboratory of Electronics, and a professor of physics.
The findings are detailed in a report published in Physical Review Letters. Guoqing Wang ’23, a recent student in Cappellaro’s lab who is now a postdoc at MIT, is the study’s first author.
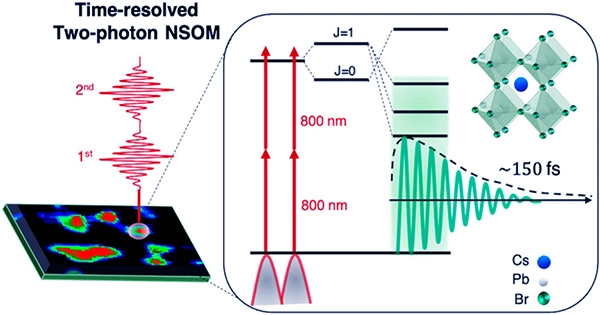
“This is one of the main problems in quantum information,” says Li. “Nuclear spin (ensembles) are particularly appealing platforms for quantum sensors, gyroscopes, and quantum memory, but in the presence of electronic spins, they have coherence times on the order of 150 microseconds… The information then simply vanishes. We’ve shown that if we understand the interactions, or noise, in these systems, we can actually do considerably better.”
Extending coherence with an ‘unbalanced echo’: The team created an approach called “unbalanced echo” to lengthen the system’s coherence duration, similar to how noise-canceling headphones use specific sound frequencies to filter out surrounding noise.
The team was able to counterbalance nuclear-electron interactions by quantifying how a specific type of noise—in this case, heat—affected nuclear quadrupole interactions in the system, increasing coherence periods from 150 microseconds to as long as 3 milliseconds.
These enhancements, though, could only be the beginning. More gains may be conceivable, according to Wang, the first author of the paper who devised the noise protection strategy, as they investigate other potential sources of noise.
“In theory, we could make it hundreds or thousands of times longer.” In practice, though, there may be additional sources of noise in the system, and we’ve demonstrated that if we can describe them, we can cancel them.”
The paper will have a “significant impact” on future work on quantum devices, according to Dmitry Budker, head of the Helmholtz Institute Mainz’s Matter-Antimatter Section and professor at Johannes Gutenberg University and the University of California, Berkeley, who was not involved in the research.
“[This group is] the world leader in the field of quantum sensing,” he claims. “They are constantly developing new approaches to spur development in this burgeoning field.” In this paper, they show how to use an inventive spin-echo approach to extend nuclear coherence duration by an order of magnitude, which should be reasonably simple to apply in applications.”
Gregory Fuchs, a professor of applied and engineering physics at Cornell University, describes the work as “innovative and impactful.”
“This [work] is significant because, while nuclear spin can theoretically have much longer coherence lifetimes than electron spins native to NV centers, no one has been able to observe long-lived nuclear spin ensembles in diamond NV center experiments,” he says. “What Professor Cappellaro and her students have demonstrated is an unexpected strategy for doing so.” This method has the potential to have a significant influence on nuclear spin ensemble applications, such as rotation sensing [a gyroscope].”
Building a sensor using ’10 billion clocks’: The paper describes experiments and calculations involving a large ensemble (approximately 10 billion) of atomic-scale impurities in diamonds known as nitrogen-vacancy centers, or NV centers, each of which exists in a specific quantum spin state for the nitrogen-14 nucleus, as well as a localized electron nearby.
While they have long been recognized as an ideal possibility for quantum sensors, gyroscopes, memory, and other applications, Wang notes that the issue has been figuring out how to enable huge ensembles of NV centers to function together.
“If you imagine each spin as a clock, these 10 billion clocks are all slightly different… “You can’t measure them all individually,” Wang explains. “What we see is that when you prepare all of these clocks, they initially sync with each other, but after a while, they completely lose their phase.” This is referred to as their de-phasing period.
“The goal is to use a billion clocks but achieve the same de-phasing time as a single clock,” he says. “This allows you to get enhancements from measuring multiple clocks while preserving phase coherence, so you don’t lose your quantum information as quickly.”
A team of researchers led by Li, Cappellaro, Wang, and other MIT graduate students originally established the underlying theory of temperature heterogeneity triggered de-phasing, which pertains to material properties, in March. That research, which appeared in the Journal of Physical Chemistry Letters, offered a theoretical approach to determining how temperature and strain affect several sorts of interactions that can lead to decoherence.
The first type of interaction, known as nuclear quadrupole interaction, happens when the nitrogen nucleus operates as an incomplete nuclear dipole—basically, as a subatomic magnet. Wang says that because the nucleus is not exactly spherical, it deforms, disturbing the dipole, which essentially interacts with itself.
Likewise, hyperfine interaction occurs when the nucleus magnetic dipole interacts with a neighboring electron magnetic dipole. Both of these sorts of interactions can vary in space and time, and de-phasing can occur when considering an ensemble of nuclear spin qubits because “clocks at different locations can get different phases.”
Based on their previous study, the scientists hypothesized that if they could quantify how heat influenced those interactions, they would be able to offset the effect and lengthen the system’s coherence durations.
“Temperature or strain affects both of those interactions,” Wang explains. “The theory we described predicted how temperature or strain would affect the quadrupole and hyperfine, and then the unbalanced echo we developed in this work is essentially canceling out the spectral drift due to one physical interaction using another different physical interaction, utilizing their correlation induced by the same noise.”
The main difference between this study and previous spin echo techniques utilized in the quantum community is that it uses distinct interaction noises to cancel each other, allowing the noises to being canceled to be highly selective. “What’s exciting, though, is that we can use this system in other ways,” he adds. “This could be used to detect temperature or strain field spatiotemporal heterogeneity.” This could be highly useful in biological systems, where even minor temperature changes can have huge impacts.”
Additional applications: Wang claims that these applications only scratch the surface of the system’s possible possibilities.
“This system could also be used to examine electrical currents in electric vehicles, and because it can measure strain fields, it could be used for non-destructive structural health evaluation,” explains Li. “Imagine a bridge; if it had these sensors, we could figure out what kind of load it’s under. Diamond sensors are already used to assess temperature distribution on the surface of materials because they are exceedingly sensitive and have a high spatial resolution.”
Another application, according to Li, could be in biology. Researchers have previously shown that using quantum sensors to map neural activity from electromagnetic fields has the potential to improve understanding of various biological processes.
The system presented in the research may also constitute a substantial advancement in quantum memory.
While some ways to prolong the coherence time of qubits for use in quantum memory exist, they are difficult and often entail “flipping”—or reversing the spin—of the NV centers. While that procedure is effective in reversing the spectral drift that produces decoherence, it also results in the loss of any information contained in the system.
By removing the requirement to reverse the spin, the new technology not only increases the coherence time of the qubits but also prevents data loss, which is a significant step forward for quantum computing.
Moving forward, the team intends to examine new sources of noise in the system, such as fluctuating electrical field interference, in order to counteract them and enhance coherence time even further.
“Now that we’ve achieved a 20-fold improvement, we’re looking at how we can improve it even more because intrinsically, this unbalanced echo can achieve an almost infinite improvement,” Li explains.
“We’re also looking into how we can apply this system to the creation of a quantum gyroscope, because coherence time is just one key parameter to building a gyroscope, and there are other parameters we’re trying to optimize to (understand) the sensitivity we can achieve compared to previous techniques.”