In a terahertz laser-equipped scanning tunneling microscope, physicists from the United States showed atomic-scale quantum sensing employing a hydrogen molecule as the quantum sensor. Scientists used a scanning tunneling microscope combined with a femtosecond terahertz laser to measure changes in the electrostatic field of a target sample, effectively transforming the hydrogen molecule into a quantum sensor.
The use of a hydrogen molecule as a quantum sensor in a terahertz laser-equipped scanning tunneling microscope, a technique that can measure the chemical properties of materials at unprecedented time and spatial resolutions, has been demonstrated by physicists at the University of California, Irvine.
This novel technique can also be used to analyze two-dimensional materials, which have the potential to play a role in advanced energy systems, electronics, and quantum computers. The researchers next stimulated the hydrogen molecule with femtosecond laser pulses, detecting changes in its quantum states at cryogenic temperatures and in the instrument’s ultrahigh vacuum environment, resulting in sub-angstrom scale, time-lapsed photographs of the sample.
As long as hydrogen can be adsorbed onto a material, you can utilize hydrogen as a sensor to characterize the material itself using electrostatic field distribution studies. The hydrogen molecule became part of the quantum microscope in the sense that everywhere the microscope scanned, there was hydrogen in between the tip and the sample.
Likun Wang
The researchers from UCI’s Departments of Physics & Astronomy and Chemistry describe how they positioned two bonded hydrogen atoms between the silver tip of the STM and a sample made of a flat copper surface arranged with small islands of copper nitride in Science today. The scientists were able to excite the hydrogen molecule and detect changes in its quantum states at cryogenic temperatures and in the ultrahigh vacuum environment of the instrument using laser pulses lasting trillionths of a second, resulting in atomic-scale, time-lapsed photographs of the sample.
“This effort represents an advancement in both the measurement technique and the scientific question that the approach enabled us to examine,” said co-author Wilson Ho, Bren Professor of physics and astronomy and chemistry. “A quantum microscope that probes the coherent superposition of states in a two-level system is far more sensitive than existing instruments that do not use this quantum physics principle.”
According to Ho, the hydrogen molecule is a two-level system because its orientation alternates between two locations, up and down and somewhat horizontally slanted. The scientists can encourage the system to transition from a ground state to an excited state in a cyclical pattern using a laser pulse, resulting in a superposition of the two states. The cyclic oscillations endure only a few tens of picoseconds, but by monitoring this “decoherence time” and the cyclic periods, the scientists were able to discern how the hydrogen molecule interacted with its surroundings.
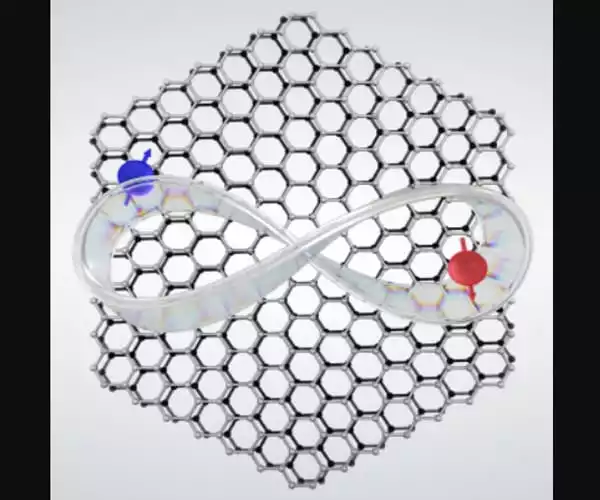
“The hydrogen molecule became part of the quantum microscope in the sense that everywhere the microscope scanned, there was hydrogen in between the tip and the sample,” Ho explained. “It creates an extraordinarily sensitive probe, allowing us to detect differences as small as 0.1 angstrom. We could see how the charge distributions changed on the sample at this resolution.”
The distance between the STM tip and the sample is nearly imperceptibly small, measuring only six angstroms or 0.6 nanometers. The STM constructed by Ho and his team is capable of detecting minute electrical currents flowing in this area and producing spectroscopic measurements demonstrating the presence of the hydrogen molecule and sample elements. According to Ho, this is the first demonstration of chemically sensitive spectroscopy using terahertz-induced rectification current across a single molecule.
According to Ho, the ability to analyze materials at this level of detail based on hydrogen’s quantum coherence can be very useful in the research and engineering of catalysts, because their functioning frequently depends on surface defects at the scale of single atoms.
“As long as hydrogen can be adsorbed onto a material, you can utilize hydrogen as a sensor to characterize the material itself using electrostatic field distribution studies,” said research lead author Likun Wang, a UCI doctoral student in physics and astronomy.