Airplane manufacturers and their government and industry customers have invested in the development of new aircraft engines that can operate at extremely high temperatures, allowing the engines to generate more energy while using less fuel. However, the extremely high temperatures can be detrimental to the engine’s materials.
One of the biggest stories of summer 2021 was post-pandemic vacation travel, which raised concerns about air travel’s contribution to greenhouse gas emissions and climate change. According to the Environmental and Energy Study Institute, commercial aviation emitted 710 million tons of global CO2 in 2013. By 2017, that figure had risen to 860 million tons, a 21% increase in four years. By 2018, it had risen to 905 million tons, accounting for 2.4 percent of total CO2 emissions.
Haydn Wadley, the Edgar Starke Professor of Materials Science and Engineering at the University of Virginia School of Engineering and Applied Science, and Jeroen Deijkers, a postdoctoral research associate in Wadley’s group, discovered a way to significantly increase the life of the materials used in these jet engines. Their paper, “A Duplex Bond Coat Approach to Environmental Barrier Coating Systems,” will be published in Acta Materialia in September 2021.
“A jet engine consumes massive amounts of air, which is compressed and mixed with hydrocarbon fuel before being burned in a combustor to power the plane’s propulsion system. The higher the temperature of the combustor, the more efficient the engine “Wadley stated.
Materials science engineers strive to reduce emissions from aircraft engines. Engineers found a way to greatly extend the life of materials used in jet engines.
Airplane engine combustion now reaches or exceeds 1500 degrees Celsius, well above the melting temperatures of engine parts typically made of nickel and cobalt alloys. Ceramics that can withstand these temperatures have been developed, but they must contend with chemical reactions caused by water vapor and unburned oxygen in the extreme combustion environment.
The ceramic of choice is silicon carbide. Engine parts made of silicon carbide, on the other hand, would only last a few thousand hours of flight time. At such high temperatures, carbon reacts with oxygen to form carbon monoxide (a gas), whereas silicon forms silica (a solid), but silica reacts with water vapor to form gaseous silicon hydroxide. In other words, the engine part gradually degrades into gas and dissipates through the tailpipe.
To protect the ceramic parts, engine manufacturers coat the silicon carbide with a two-layer coating known as an environmental barrier coating system. The outer layer is intended to slow the spread of oxygen and water vapor toward the silicon carbide during flight, while an inner silicon bond coat protects the surface of the silicon carbide by reacting with the oxygen to form a thin layer of silica. However, there are still issues with this design.
“The time it takes for the silica layer thickness to reach a critical point where the stress caused by expansion and contraction during repeated heating and cooling causes the coating to pop off,” Wadley explained.
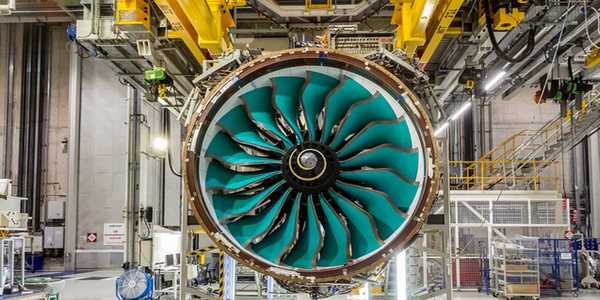
Scientists and engineers have devised two basic strategies for delaying the separation of the coating and extending the life of expensive engine components. They can increase the thickness of the outer coating layer to slow the arrival of oxygen at the bond coat, but this adds weight and cost. Alternatively, they can create a different type of protective oxide that does not “pop-off.”
The second strategy was pursued by Deijkers and Wadley. Their solution employs an outer layer of ytterbium disilicate, a rare earth element with the same thermal expansion characteristics as silicon and silicon carbide but is slow to transport oxygen and water vapor toward the silicon layer. They began by depositing the silicon bond coat, followed by a thin layer of hafnium oxide between the silicon and the ytterbium disilicate outer layer.
Their experiments show that when silica forms on silicon, it immediately reacts with hafnia to form silicon-hafnium oxide or hafnon. The thermal expansion and contraction of hafnon are the same as those of the rest of the coating and will never cause the coating to pop off or crack. Wadley refers to it as “hafnia fairy dust.”
“When we deposit a very thin layer of hafnia on top of silicon, followed by a layer of ytterbium disilicate,” Deijkers explained, “the oxygen that passes through the ytterbium disilicate creates a chemical reaction with the underlying materials to form the hafnon.”
This breakthrough in environmental barrier coatings was made possible by Deijkers’ access to unique equipment in Wadley’s lab, specifically a directed vapor deposition system. The ability to deposit a ytterbium disilicate film thinner than the width of a human hair is critical to their success.
A powerful 10-kilowatt focused electron beam is used in the directed vapor deposition process to melt material in a low-pressure chamber. The vapor is transported by a supersonic gas jet to the silicon-coated silicon carbide, where it condenses to form a thin film. They then deposit the final ytterbium disilicate layer using a plasma spray method, and the coated component is ready for testing.
In October 2020, Deijkers successfully defended his dissertation, combining his interests in aircraft and high-temperature materials for his Ph.D. research and following in his father’s footsteps into materials science and engineering.
“My father used to work on dredges. I got the engineering bug after seeing the pump house glow orange-white in the furnace “According to Deijkers. Deijkers, who is Dutch, combined these childhood memories with his desire to serve in the Dutch Air Force, earning a bachelor’s and master’s degree in aerospace engineering from the Delft University of Technology.
When Deijkers began applying to Ph.D. programs in the United States, Wadley was drawn to his master’s thesis on thermal barrier coatings. Deijkers’ arrival couldn’t have come at a better time. Brad Richards, a group member who received his Ph.D. in materials science and engineering from UVA in 2015, developed a silicon-ytterbium disilicate coating system for ceramics that was later discovered to be very similar to that used by aircraft engine manufacturers.
Deijkers’ dissertation improves Richards’ coating system by increasing understanding of the surface chemistry involved and making the coating system more commercially viable. “One set of questions that drove my research focused on how long it takes for the hafnon to form during the oxidation process,” Deijkers explained. “I wanted to know how this process really works and whether we could actually use it.”
Today’s methods are rooted in deposition techniques developed in the 1970s.
“Our research significantly improves on the state-of-the-art in industry,” Deijkers said. “According to my rough estimate, if industrial manufacturers were able to implement these newer processing techniques, they could increase the lifetime of engine parts by up to 200 times. However, there are numerous obstacles to overcome in order to achieve that level of performance.”
Wadley’s research team made these advances with the help of the Office of Naval Research, which awarded Wadley’s team two consecutive grants over a six-year period.
“The problems we face are multidisciplinary and multi-institutional,” Wadley explained. “To make progress, we must combine knowledge from mechanics, chemistry, and materials science. Aside from the immediate need to reduce CO2 emissions from propulsion technology, our research contributes to the global transition from carbon-containing hydrocarbons to hydrogen fuels and, eventually, the electrification of air travel platforms.”
While Deijkers hopes to attract private industry to the team’s coatings system and deposition process, his long-term career goal is to conduct scientific research at a national laboratory or in academia. “The country is in desperate need of talent in this area,” Wadley said. “We are desperately in need of bright, creative people who want to be trained to solve these kinds of problems for society in the future.”
Deijkers encourages UVA Engineering undergraduates to participate in the interdisciplinary research being conducted in Wadley’s group, just as he did with Richards’ research. “We had students from aerospace engineering, physics, and systems engineering,” Deijkers explained. “We are working on a variety of aspects of the problem, including computer modeling, materials synthesis, and thermo-mechanical life design. We always have things for undergraduates to do, and we welcome them to conduct research with us.”