A group of researchers has created a new class of biomaterial inks that mimic the native properties of highly conductive human tissue, similar to skin, and are required for the ink to be used in 3D printing.
Sensors, actuators, microfluidics, and electronics can now be designed on flexible, conformal, and/or stretchable sublayers for wearable, implantable, or ingestible applications. These devices, however, have very different mechanical and biological properties than human tissue and thus cannot be integrated into the human body.
A team of Texas A&M University researchers has developed a new class of biomaterial inks that mimic the native characteristics of highly conductive human tissue, similar to skin, which are required for the ink to be used in 3D printing.
This biomaterial ink makes use of molybdenum disulfide, a new class of 2D nanomaterial (MoS2). MoS2’s thin-layered structure contains defect centers, which make it chemically active when combined with modified gelatin to form a flexible hydrogel similar to the structure of Jell-O.
These 3D-printed devices are extremely elastomeric and can be compressed, bent, or twisted without breaking. Moreover, because these devices are electronically active, they can monitor dynamic human motion and pave the way for continuous motion monitoring.
Kaivalya Deo
“The impact of this work is far-reaching in 3D printing,” said Dr. Akhilesh Gaharwar, associate professor in the Department of Biomedical Engineering and Presidential Impact Fellow. “This newly designed hydrogel ink is highly biocompatible and electrically conductive, paving the way for the next generation of wearable and implantable bioelectronics.”
This study was recently published in ACS Nano.
Because the ink has shear-thinning properties that cause viscosity to decrease as force increases, it is solid inside the tube but flows more like a liquid when squeezed, similar to ketchup or toothpaste. The researchers combined these electrically conductive nanomaterials with modified gelatin to create a hydrogel ink with properties critical for designing ink for 3D printing.
“These 3D-printed devices are extremely elastomeric and can be compressed, bent, or twisted without breaking,” said Kaivalya Deo, the paper’s lead author and a graduate student in the biomedical engineering department. “Moreover, because these devices are electronically active, they can monitor dynamic human motion and pave the way for continuous motion monitoring.”
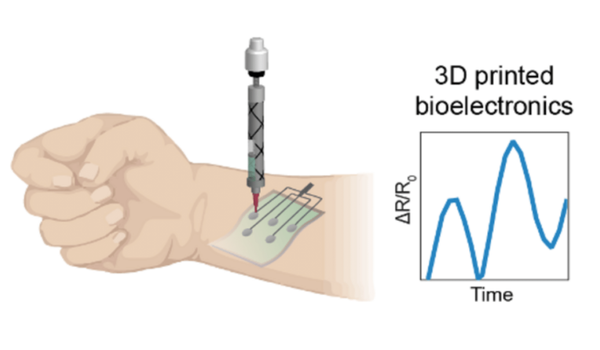
In order to 3D print the ink, researchers in the Gaharwar Laboratory designed a cost-effective, open-source, multi-head 3D bioprinter that is fully functional and customizable, running on open-source tools and freeware. This also allows any researcher to build 3D bioprinters tailored to fit their own research needs.
The electrically conductive 3D-printed hydrogel ink can create complex 3D circuits and is not limited to planar designs, allowing researchers to create customizable bioelectronics tailored to the needs of individual patients.
Deo was able to print electrically active and stretchable electronic devices using these 3D printers. These devices have exceptional strain sensing capabilities and can be used to design customizable monitoring systems. This also opens up new avenues for the development of stretchable sensors with integrated microelectronic components.
One of the potential applications of the new ink is in 3D printing electronic tattoos for patients with Parkinson’s disease. Researchers envision that this printed e-tattoo can monitor a patient’s movement, including tremors.
Elastomeric and conductive biointerfaces with native tissue-like mechanical properties are required for flexible electronics. Traditional approaches to engineering such a biointerface frequently combine conductive nanomaterials with polymeric hydrogels that are cross-linked using toxic photoinitiators. Furthermore, under physiological conditions, these systems frequently exhibit poor biocompatibility and face trade-offs between conductivity and mechanical stiffness.
The National Institute of Biomedical Imaging and Bioengineering, the National Institute of Neurological Disorders and Stroke, and the Texas A&M University President’s Excellence Fund all contributed to this research. In collaboration with the Texas A&M Engineering Experiment Station, a provisional patent on this technology has been filed.