Scientists are striving to develop more efficient and greener methods to power the world as climate change continues to wreak havoc on our planet. Biological systems might be an enticing alternative to typical petrochemical processes that produce substantial greenhouse emissions and other waste items.
Any of a broad set of chemicals (as opposed to fuels) produced from petroleum and natural gas and utilized for a variety of commercial applications is referred to as a petrochemical. However, the term has been expanded to encompass all aliphatic, aromatic, and naphthenic organic compounds, as well as carbon black and inorganic elements such as sulfur and ammonia.
Michael Jewett of Northwestern Engineering and academics from the University of Texas at Austin have recently collaborated to improve our understanding of biochemical processes and boost the rate at which biological systems produce chemicals. The discoveries might help us get closer to establishing long-term alternatives to oil-based materials, fuels, and other items.
Many of the petrochemicals can also be produced from other sources, such as coal, coke, or vegetable products. The remainder is generally made up of coal and biofuels. The petrochemical plant is a key, if not the most important, component of the industry. Chemical feedstock is any sort of unprocessed substance that is used as a basic material in a manufacturing process before being converted into another end product.
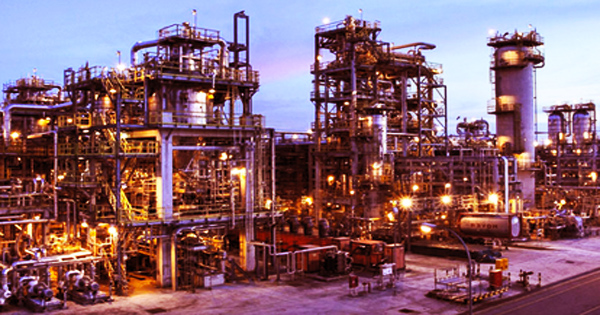
The paper “An Integrated In Vivo/In Vitro Framework to Enhance Cell-Free Biosynthesis with Metabolically Rewired Yeast Extracts,” published Aug. 26 (2021) in the journal Nature Communications, describes the development of optimized in vitro biosynthesis (biochemical production) processes that use cell extracts from engineered strains of Saccharomyces cerevisiae (brewer’s yeast).
Blake Rasor, a PhD student in Jewett’s lab, co-authored the study alongside Jewett, the Walter P. Murphy Professor of Chemical and Biological Engineering at the McCormick School of Engineering. The experiment was carried out in conjunction with Hal Alper’s research group at the University of Texas at Austin.
The work was funded by the Emerging Technologies Opportunity Program of the US Department of Energy Joint Genome Institute (ETOP). The ETOP provides funds to help researchers throughout the world create innovative technologies by utilizing JGI’s user programs to enhance energy and environmental applications.
S. cerevisiae is a highly controlled framework for biochemical synthesis, thanks to decades of metabolic investigations and genetic tool development. This yeast has been designed to generate countless target compounds utilized in industrial and medicinal purposes, in addition to its traditional uses in baking and brewing.
Cellular production systems, on the other hand, are torn between producing additional cells and producing the designed output. Breaking the biological machinery out of cells and utilizing the extracted material for cell-free biochemical processes allows Jewett’s group to bypass these growth and survival restrictions, allowing for the optimization of levers that are difficult to tune in living cells.
Previously, unaltered E. coli strains were utilized in cell-free biosynthetic experiments with crude cell extracts. The researchers broadened the breadth of this method by integrating S. cerevisiae extracts and cellular metabolic engineering methods to boost the biosynthetic potential of cell-free processes. This shows that metabolic rewiring in cells results in extracts and cell cultures with higher volumetric outputs than wildtype (unchanged) extracts and cell cultures.
The ability to produce three chemical products (butanediol, glycerol, and itaconic acid) at a pace up to ten times quicker than cellular methods demonstrates the versatility and usefulness of combining cellular engineering and cell-free biosynthesis.
“This could expand the breadth of biological platforms underpinning efforts in sustainability,” Rasor said.
“Our work further joins an emerging area of science that seeks to use cell-free systems from crude cell extracts for designing cellular function, on-demand biomanufacturing, and portable diagnostics,” said Jewett, director of the Center for Synthetic Biology. “Indeed, in order to establish a sustainable bioeconomy, these activities are broadening the scope of biomanufacturing.”
Next stages, according to Jewett and his coworkers, include route prototyping in the setting of changing metabolism, as well as cell-free biomanufacturing to supplement existing cell-based methods.
“Expanding the integrated cell/cell-free metabolic engineering strategy to yeast strains producing other value-added biochemical products and increasing the scale of cell-free reactions could spearhead the development of sustainable, economically viable alternatives to current chemical production processes,” he said.