Researchers were successful in changing not only the strength but also the nature of the interaction between microscopic quantum magnets known as spins. Instead of becoming completely disordered, the specially prepared magnets can retain their original orientation for an extended period of time. The physicists have successfully demonstrated programmable control of spin interactions in isolated quantum systems with these findings.
Nature’s interactions determine the forces that exist between particles, atoms, molecules, and even macroscopic objects such as magnets. Two closely spaced bar magnets, for example, realign themselves as a result of magnetic forces.
A team led by Prof. Dr. Matthias Weidemüller and Dr. Gerhard Zürn at Heidelberg University’s Center for Quantum Dynamics has now succeeded in changing not only the strength but also the nature of the interaction between microscopic quantum magnets known as spins. Instead of becoming completely disordered, the specially prepared magnets can retain their original orientation for an extended period of time. The Heidelberg physicists have successfully demonstrated programmable control of spin interactions in isolated quantum systems with these findings.
An ensemble of Rydberg atoms exhibits exactly the same properties as interacting disordered quantum magnets, making it an ideal platform for simulating and exploring quantum magnetism.
Dr. Nithiwadee Thaicharoen
When magnetic systems are prepared in an unstable configuration, they can exhibit unexpected behavior. For example, forcing a collection of spatially disordered magnetic dipoles, such as bar magnets, to align in the same direction will result in magnet reorientation. This eventually leads to an equilibrium in which all magnets are oriented randomly. While most studies used to be limited to classical magnetic dipoles, it has recently become possible to extend approaches to quantum magnets using what are known as quantum simulators. Synthetic atomic systems mimic the fundamental physics of magnetic phenomena in a highly controlled environment where all relevant parameters can be adjusted almost at will.
The researchers used gas of atoms cooled to near absolute zero temperatures in their quantum simulation experiments. The atoms were excited to extremely high electronic states using laser light, separating the electron from the atomic nucleus by almost macroscopic distances. These “atomic giants,” also known as Rydberg atoms, interact with one another over almost a hair’s breadth distances. “An ensemble of Rydberg atoms exhibits exactly the same properties as interacting disordered quantum magnets, making it an ideal platform for simulating and exploring quantum magnetism,” says Dr. Nithiwadee Thaicharoen, who was a postdoc on Prof. Weidemüller’s team at the Institute for Physics and is now a professor in Thailand.
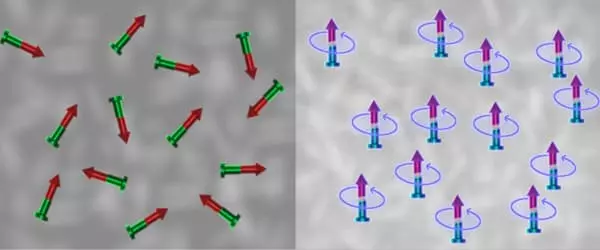
The Heidelberg physicists’ key trick was to manipulate the dynamics of quantum magnets using methods from nuclear magnetic resonance. The researchers use specially designed periodic microwave pulses to modify the atomic spin in their experiments. The precise control of the interaction between the atomic spins using this technique, known as Floquet engineering, was a major challenge. “The microwave pulses had to be applied to the Rydberg atoms at billionth-of-a-second timescales, with these atoms being super-sensitive to any external perturbation, however tiny, like minute electric fields,” explains Dr. Clément Hainaut, a postdoc on the team who recently moved to the University of Lille (France).
“Through our control protocol, we were able to halt the spin’s seemingly inevitable reorientation and maintain a macroscopic magnetization,” explains doctoral student Sebastian Geier. “Using our Floquet engineering approach, we should now be able to reverse the timeline so that the spin system inverts its evolution after a very complex dynamic. It would be like a shattered glass magically reassembling itself after collapsing on the floor.”
The research is a significant step toward a better understanding of fundamental processes in complex quantum systems. “After the first and second quantum revolutions, which led to the understanding of systems and the precise control of single objects, we are confident that our technique of dynamically adjusting interactions in a programmable fashion opens a path to Quantum Technologies 3.0,” says Matthias Weidemüller, professor at Heidelberg University’s Institute for Physics and Director of the Center for Quantum Dynamics.
The experiments were carried out within the framework of Heidelberg University’s STRUCTURES Cluster of Excellence and the “Isolated quantum systems and universality under extreme conditions” Collaborative Research Centre (ISOQUANT). The activities are also part of the PASQuans collaboration, which stands for “Programmable Atomic Large-Scale Quantum Simulation” and is part of the European Quantum Technologies Flagship.