The magnetic field of Earth is far stronger than that of Venus or Mars, which explains why life on Earth is abundant while its nearest neighbors do not currently support life. The Earth’s magnetic field extends from its outer core (where swirling molten iron produces a dynamo effect) up through the magnetosphere and out into space for thousands of miles. This magnetic field shields the Earth from solar wind and cosmic rays, which would otherwise destroy the upper atmosphere, including the ozone layer, which protects us from lethal ultraviolet radiation. Mars and Venus are not so safe.
Scientists describe a method for studying smaller magnetospheres in the laboratory, which can be as thin as millimeters. The Large Plasma Device’s magnetic field is combined with a fast laser-driven plasma and a current-driven dipole magnet in the new experimental platform. The LAPD magnetic field represents the interplanetary magnetic field of the solar system, whereas the laser-driven plasma represents the solar wind and the dipole magnet represents the Earth’s inherent magnetic field. Motorized probes can perform three-dimensional system scans by combining data from tens of thousands of laser shots.
A magnetosphere forms around any magnetized object, such as a planet, that is immersed in a stream of ionized gas known as plasma. Because Earth has an intrinsic magnetic field, it is surrounded by a large magnetosphere that extends into space, blocks lethal cosmic rays and particles from the sun and stars, and allows life to exist.
We developed a new experimental platform to study mini-magnetospheres on the Large Plasma Device (LAPD) at UCLA to overcome these limitations. The magnetic field of the LAPD is combined with a fast laser-driven plasma and a current-driven dipole magnet on this platform.
Derek Schaeffer
Scientists from Princeton, UCLA, and Portugal’s Instituto Superior Técnico report a method for studying smaller magnetospheres, sometimes just millimeters thick, in the laboratory in Physics of Plasmas, published by AIP Publishing.
These mini-magnetospheres, which have been observed around comets and near certain regions of the moon, have been proposed as a means of propulsion for spacecraft. They serve as excellent research platforms for larger planet-sized magnetospheres. Previously, mini-magnetospheres were created in the laboratory using plasma wind tunnels or high-energy lasers. However, previous experiments were limited to 1D magnetic field measurements, which do not capture the full 3D behavior that scientists require.
“We developed a new experimental platform to study mini-magnetospheres on the Large Plasma Device (LAPD) at UCLA to overcome these limitations,” said author Derek Schaeffer. The magnetic field of the LAPD is combined with a fast laser-driven plasma and a current-driven dipole magnet on this platform.
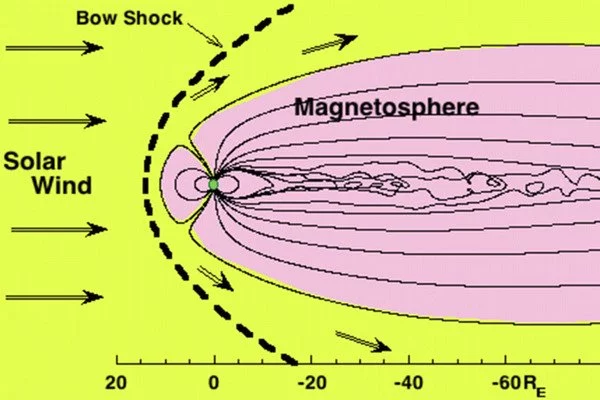
The LAPD magnetic field simulates the solar system’s interplanetary magnetic field, while the laser-driven plasma simulates solar wind and the dipole magnet simulates the Earth’s inherent magnetic field. By combining data from tens of thousands of laser shots, motorized probes enable three-dimensional system scans.
One advantage of using this setup is that the magnetic field and other parameters can be carefully varied and controlled. All signs of a magnetosphere vanish when the dipole magnet is turned off. When the dipole’s magnetic field is activated, a magnetopause can be detected, which is crucial evidence of the formation of a magnetosphere.
A magnetopause is a point in the magnetosphere where the pressure from the planetary magnetic field is perfectly balanced by the solar wind. The experiments revealed that as the dipole magnetic field is increased, the magnetopause grows larger and stronger.
The effect on the magnetopause was predicted by computer simulations, which the investigators used to better understand and validate their experimental results. These simulations will also guide future experiments, such as those involving a cathode recently installed on the LAPD.
“The new cathode will enable faster plasma flows, which in turn will allow us to study the bow shocks observed around many planets,” Schaeffer said. Other experiments will study magnetic reconnection, an important process in Earth’s magnetosphere in which magnetic fields annihilate to release tremendous energy.
The magnetopause is the boundary between the solar wind and the Earth’s magnetic field. As the Earth is buffeted by the ever-changing solar wind, the boundary is constantly in motion. While the magnetopause protects us from the solar wind to some extent, it is not impenetrable, and energy, mass, and momentum are transferred from the solar wind to regions within Earth’s magnetosphere. The interaction of the solar wind and the Earth’s magnetic field, as well as the influence of the underlying atmosphere and ionosphere, results in the formation of various regions of fields, plasmas, and currents within the magnetosphere, such as the plasmasphere, the ring current, and radiation belts.