Certain types of clay minerals have the ability to capture carbon dioxide (CO2) from the atmosphere or from industrial processes. This process is known as mineral carbonation, and it involves the reaction of CO2 with metal oxide minerals in the presence of water to form stable carbonates.
Clay minerals that are particularly effective at carbon capture include serpentine, olivine, and zeolite. Serpentine is a magnesium silicate mineral that can capture large amounts of CO2, while olivine is an iron-magnesium silicate mineral that reacts rapidly with CO2 to form stable magnesium carbonate. Zeolite is a type of aluminosilicate mineral that has a high surface area and can adsorb CO2 onto its surface.
Scientists have been studying how a type of clay can absorb and store carbon dioxide using powerful computer models and laboratory experiments. Carbon dioxide, a gas that traps heat and contributes to climate change, is nearly double what it was before the Industrial Revolution, but it only accounts for 0.0415% of the air we breathe.
This presents a challenge to researchers working on artificial trees or other methods of directly capturing carbon dioxide from the air. A team of scientists led by Sandia National Laboratories is attempting to solve this problem.
The team, led by Sandia chemical engineer Tuan Ho, has been studying how a type of clay can absorb and store carbon dioxide using powerful computer models and laboratory experiments. The researchers published their preliminary findings in The Journal of Physical Chemistry Letters earlier this week.
“These fundamental findings have the potential for direct-air capture, which is what we’re working toward,” said Ho, the paper’s lead author. “Clay is extremely cheap and abundant in nature. If this high-risk, high-reward project results in technology, we should be able to significantly reduce the cost of direct-air carbon capture.”
We want low-cost energy without destroying the environment. We can live in a way that reduces carbon dioxide emissions, but we have no control over what our neighbors do. Direct-air carbon capture is critical for reducing the amount of CO2 in the atmosphere and mitigating the CO2 emissions of our neighbors.
Susan Rempe
Why capture carbon?
Carbon capture and sequestration is the process of removing excess carbon dioxide from the atmosphere and storing it deep underground in order to mitigate the effects of climate change, such as more frequent severe storms, rising sea levels, and increased droughts and wildfires. This carbon dioxide could be captured from fossil-fuel-burning power plants, other industrial facilities such as cement kilns, or directly from the air, which would be more difficult technologically. Carbon capture and sequestration is widely regarded as one of the least contentious climate-intervention technologies.
“We want low-cost energy without destroying the environment,” said Susan Rempe, a Sandia bioengineer and project senior scientist. “We can live in a way that reduces carbon dioxide emissions, but we have no control over what our neighbors do. Direct-air carbon capture is critical for reducing the amount of CO2 in the atmosphere and mitigating the CO2 emissions of our neighbors.”
Ho envisions clay-based devices being used as sponges to absorb carbon dioxide, and then the carbon dioxide being “squeezed” out of the sponge and pumped deep underground. Alternatively, the clay could be used as a filter to capture and store carbon dioxide from the air.
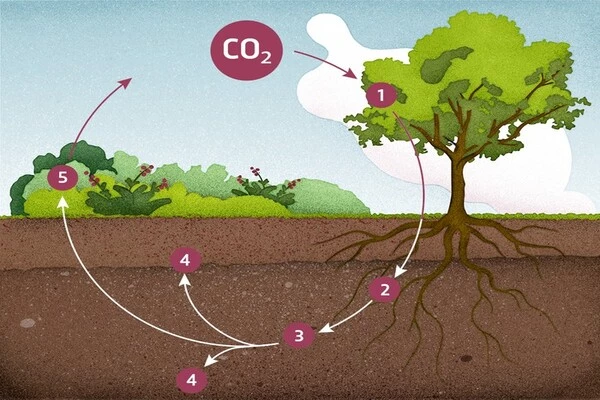
In addition to being cheap and widely available, clay is also stable and has a high surface area – it is comprised of many microscopic particles that in turn have cracks and crevasses about a hundred thousand times smaller than the diameter of a human hair. These tiny cavities are called nanopores, and chemical properties can change within these nanoscale pores, Rempe said.
Rempe has previously investigated nanostructured materials for carbon dioxide capture. She is, in fact, a member of a team that investigated a biological catalyst for converting carbon dioxide into water-stable bicarbonate, designed a thin, nanostructured membrane to protect the biological catalyst, and was granted a patent for their bio-inspired, carbon-catching membrane. Of course, this membrane is not made of cheap clay, and it was originally designed to work in fossil-fuel-burning power plants or other industrial facilities, according to Rempe.
“These are two complementary possible solutions to the same problem,” she said.
How to simulate the nanoscale?
Molecular dynamics is a kind of computer simulation that looks at the movements and interactions of atoms and molecules at the nanoscale. By looking at these interactions, scientists can calculate how stable a molecule is in a particular environment – such as in clay nanopores filled with water.
“Molecular simulation is really a powerful tool to study interactions at the molecular scale,” Ho said. “It allows us to fully understand what is going on among the carbon dioxide, water and clay, and the goal is to use this information to engineer a clay material for carbon-capture applications.”
In this case, Ho’s molecular dynamics simulations revealed that carbon dioxide can be much more stable in wet clay nanopores than in plain water. This is due to the fact that the atoms in water do not evenly share their electrons, causing one end to be slightly positively charged and the other to be slightly negatively charged. The atoms in carbon dioxide, on the other hand, share their electrons evenly, and like oil mixed with water, carbon dioxide is more stable near similar molecules, such as the silicon-oxygen regions of the clay, according to Rempe.
Purdue University researchers led by Professor Cliff Johnston recently used experiments to confirm that water confined in clay nanopores absorbs more carbon dioxide than plain water, according to Ho.
According to Ho, Sandia postdoctoral researcher Nabankur Dasgupta discovered that inside the oil-like regions of the nanopores, it takes less energy to convert carbon dioxide into carbonic acid, making the reaction more favorable than the same conversion in plain water. By making this conversion more favorable and requiring less energy, the oil-like regions of clay nanopores ultimately allow for more carbon-dioxide capture and storage, he added.
“So far, this indicates that clay is a good material for capturing carbon dioxide and converting it into another molecule,” Rempe said. “And we know why, so that the synthesisers and engineers can improve the material. The simulations can also be used to guide experiments to test new hypotheses.”