Using common microprocessor manufacturing methods, researchers create transistors by combining silicon with biological silk. To change its properties, the silk protein can be easily modified with other chemical and biological molecules, resulting in circuits that respond to biology and the environment.
Your phone’s microprocessor chips may contain more than 15 billion tiny transistors. Transistors are made of silicon, metals such as gold and copper, and insulators that take an electric current and convert it to 1s and 0s to communicate and store information. The materials used in transistors are inorganic, derived primarily from rock and metal.
But what if you could make these fundamental electronic components biological, allowing them to respond to their surroundings and change like living tissue?
This is what a team at Tufts University Silklab did when they created transistors using biological silk as the insulating material. They published their findings in the journal Advanced Materials.
You could imagine creating circuits that make use of information that is not represented by the discrete binary levels used in digital computing, but can process variable information as in analog computing, with the variation caused by changing what’s inside the silk insulator.
Fiorenzo Omenetto
The structural protein of silk fibers, silk fibroin, can be precisely deposited onto surfaces and easily modified with other chemical and biological molecules to change its properties. Silk that has been functionalized in this way can pick up and detect a variety of components from the body or environment.
The team’s first prototype device used hybrid transistors to create a highly sensitive and ultrafast breath sensor that detected changes in humidity. Further modifications to the silk layer may allow devices to detect certain cardiovascular and pulmonary diseases, as well as sleep apnea, or to detect carbon dioxide levels and other gases and molecules in the breath that may provide diagnostic information. They could potentially provide information on oxygenation and glucose levels, circulating antibodies, and more when combined with blood plasma.
Prior to the development of the hybrid transistors, the Silklab, led by Fiorenzo Omenetto, the Frank C. Doble Professor of engineering, had already used fibroin to make bioactive inks for fabrics that can detect changes in the environment or on the body, sensing tattoos that can be placed under the skin or on the teeth to monitor health and diet, and sensors that can be printed on any surface to detect pathogens like the virus responsible for COVID19.
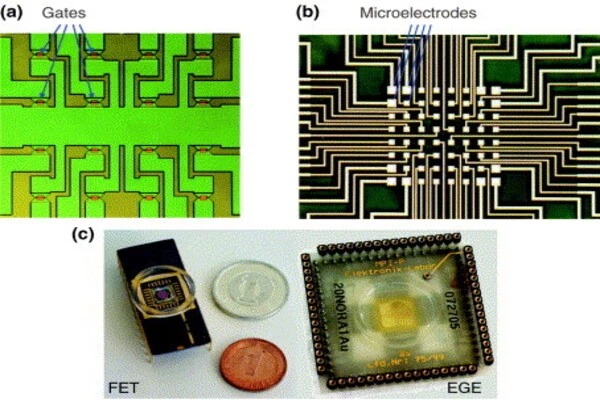
How It Works
A transistor is nothing more than an electrical switch with one metal electrical lead coming in and another going out. The semiconductor material is sandwiched between the leads because it cannot conduct electricity unless coaxed.
An insulator separates another source of electrical input, known as a gate, from everything else. The gate serves as the “key” for turning on and off the transistor. It activates the on-state when a threshold voltage–let’s call it “1”–creates an electric field across the insulator, priming electron movement in the semiconductor and initiating current flow through the leads.
In a biological hybrid transistor, a silk layer is used as the insulator, and when it absorbs moisture, it acts like a gel carrying whatever ions (electrically charged molecules) are contained within. The gate triggers the on-state by rearranging ions in the silk gel. By changing the ionic composition in the silk, the transistor operation changes, allowing it to be triggered by any gate value between zero and one.
“You could imagine creating circuits that make use of information that is not represented by the discrete binary levels used in digital computing, but can process variable information as in analog computing, with the variation caused by changing what’s inside the silk insulator,” said Omenetto. “This opens up the possibility of introducing biology into computing within modern microprocessors,” said Omenetto. Of course, the most powerful known biological computer is the brain, which processes information with variable levels of chemical and electrical signals.
The technical challenge in developing hybrid biological transistors was to achieve silk processing at the nanoscale, down to 10nm, or less than 1/10000th the diameter of a human hair. “Now that we’ve done that, we can make hybrid transistors using the same fabrication processes that are used for commercial chip manufacturing,” said Beom Joon Kim, a postdoctoral researcher at the School of Engineering. “This means you can make a billion of these with capabilities available today.”
Having billions of transistor nodes with connections reconfigured by biological processes in the silk could lead to microprocessors that act like neural networks used in artificial intelligence. “Looking ahead, one could imagine having integrated circuits that train themselves, respond to environmental signals, and record memory directly in the transistors rather than sending it to separate storage,” he said.
Large-scale analog and neuromorphic computing, as well as devices capable of detecting and responding to more complex biological states, remain undeveloped. Omenetto is optimistic about the future. “This opens up a new way of thinking about the interface between electronics and biology, with many important fundamental discoveries and applications ahead.”