A wearable skin patch that monitors hemoglobin in deep tissues would use a technology called transdermal optical spectroscopy (TOS) to non-invasively measure the concentration of hemoglobin in the blood vessels located just beneath the skin. These patches are typically small, flexible, and easy to wear, and they can provide continuous monitoring of hemoglobin levels over time. The data can then be transmitted wirelessly to a device or computer for analysis. Some use case scenarios are monitoring anemia, disease, or hydration in patients or athlete.
An engineering team at the University of California, San Diego, has created an electronic patch capable of monitoring biomolecules in deep tissues, including hemoglobin. This provides medical professionals with unprecedented access to critical information that could aid in the detection of potentially fatal conditions such as malignant tumors, organ dysfunction, cerebral or gut hemorrhages, and more.
“The amount and distribution of hemoglobin in the body provide important information about blood perfusion or accumulation in specific locations. Our device has great potential for close monitoring of high-risk groups, allowing for timely interventions in critical situations “Sheng Xu, a UC San Diego professor of nanoengineering and the study’s corresponding author, explained.
The paper, “A photoacoustic patch for three-dimensional imaging of hemoglobin and core temperature,” is published in the issue of Nature Communications.
Continuous monitoring is critical for timely interventions to prevent life-threatening conditions from worsening quickly. Wearable devices based on electrochemistry for biomolecules detection, not limited to hemoglobin, are good candidates for long-term wearable monitoring applications.
Xiangjun Chen
Low blood perfusion inside the body can cause severe organ dysfunction and is linked to a variety of ailments, including heart attacks and vascular diseases of the extremities. At the same time, abnormal blood accumulation in areas such as the brain, abdomen, or cysts can indicate cerebral or visceral hemorrhage or malignant tumors. Continuous monitoring can help diagnose these conditions and facilitate timely, potentially life-saving interventions.
The new sensor overcomes some significant limitations in existing biomolecule monitoring methods. Magnetic resonance imaging (MRI) and X-ray computed tomography rely on bulky equipment that can be difficult to obtain and typically only provide information on the immediate status of the molecule, making them unsuitable for long-term biomolecule monitoring.
“Continuous monitoring is critical for timely interventions to prevent life-threatening conditions from worsening quickly,” said Xiangjun Chen, a nanoengineering Ph.D. student in the Xu group and study co-author. “Wearable devices based on electrochemistry for biomolecule detection, not limited to hemoglobin, are good candidates for long-term wearable monitoring applications. However, the existing technologies only achieve the ability of skin-surface detection.”
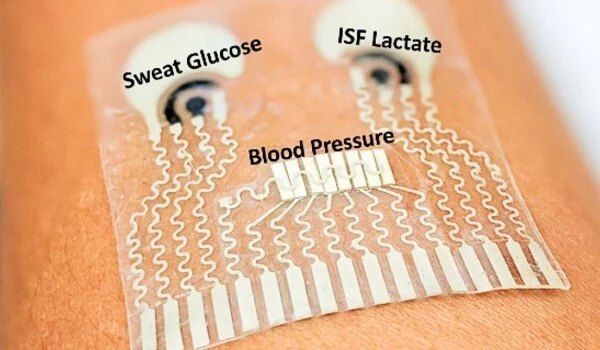
The new, flexible, low-form-factor wearable patch adheres to the skin comfortably, allowing for noninvasive long-term monitoring. In contrast to other wearable electrochemical devices that only sense biomolecules on the skin’s surface, it can perform three-dimensional mapping of hemoglobin with a submillimeter spatial resolution in deep tissues, down to centimeters below the skin. It has a high contrast with other tissues. Because of its optical selectivity, it can broaden the range of detectable molecules by incorporating different laser diodes with different wavelengths, in addition to its potential clinical applications.
The patch is equipped with arrays of laser diodes and piezoelectric transducers in its soft silicone polymer matrix. Laser diodes emit pulsed lasers into the tissues. Biomolecules in the tissue absorb the optical energy, and radiate acoustic waves into surrounding media.
“Piezoelectric transducers receive the acoustic waves, which are processed in an electrical system to reconstruct the spatial mapping of the wave-emitting biomolecules,” said Xiaoxiang Gao, a postdoctoral researcher in Xu’s lab and co-author of the study.
“With its low-power laser pulses, it is also much safer than X-ray techniques that have ionizing radiation,” said Hongjie Hu, a postdoctoral researcher in the Xu group and study coauthor.
Based on its early success, the team intends to expand the device’s flexibility and potential clinical utility by shrinking the backend controlling system to a portable-sized device for laser diode driving and data acquisition.
They also intend to investigate the wearable’s capability for core temperature monitoring. “Because the amplitude of the photoacoustic signal is proportional to temperature, we have demonstrated core temperature monitoring on ex-vivo experiments,” Xu explained. “However, to validate the core temperature monitoring on the human body, interventional calibration is required.”