Scientists studying the unusual movement of electrons in graphene have discovered a new understanding of the physics of conductive materials. Because of its two-dimensional structure, graphene is many orders of magnitude more conductive than copper. Most metals’ conductivity is limited by crystal imperfections, which cause electrons to scatter like billiard balls as they move through the material.
Light was used to bend electrons in bilayer graphene, according to the researchers. The flow of electrons in materials determines their electronic properties. When a voltage is applied to a conducting material, for example, electrons begin to flow, resulting in an electrical current. These electrons are commonly thought to move in straight lines along the electric field, similar to a ball rolling down a hill. However, these are not the only paths electrons can take: when a magnetic field is applied, electrons no longer travel in straight lines along the electric field, but bend instead. The twisted electronic flows produce transverse signals known as “Hall” responses.
Now, is it possible to bend electrons without applying a magnetic field? In a study recently published in Science, an international team of researchers report that circular polarized light can induce bent electronic flows in bilayer graphene. The study has been carried out by a team including ICFO scientists Jianbo Yin (currently researcher from the Beijing Graphene Institute, China), David Barcons, Iacopo Torre, led by ICREA Prof. at ICFO Frank Koppens, in collaboration with Cheng Tan and James Hone from Columbia University, Kenji Watanabe and Takashi Taniguchi from NIMS Japan and Prof. Justin Song from Nanyang Technological University (NTU) in Singapore.
We can control the bending of the electrons with the out-of-plane electric field we apply. The Hall conductivity measures how much we can change the bending angle of these electrons. The Berry curvature can be tuned by adjusting the voltage ‘knob,’ leading to massive Hall conductivity.
Jianbo Yin
Jianbo Yin, the study’s first author, recalls how it all started. “This collaborative study began in 2016 with a conversation between Justin Song and Frank Koppens at a scientific conference.” “Electrons are not just particles,” says Justin Song, “but can have a quantum wave-like nature.” The wave pattern of electrons in quantum materials, such as bilayer graphene, can exhibit a complex winding, which is often referred to as quantum geometry. “Frank and I discussed the possibility of using quantum geometry in bilayer graphene to bend the flow of electrons with light rather than magnetic fields.”
With this in mind, Jianbo Yin, a researcher on Frank Koppens’ team, decided to take on the challenge of reproducing this unusual phenomenon experimentally. “Our device was extremely difficult to construct. To improve device quality, it took many devices and a trip to Columbia University to work with Cheng Tan and James Hone.”
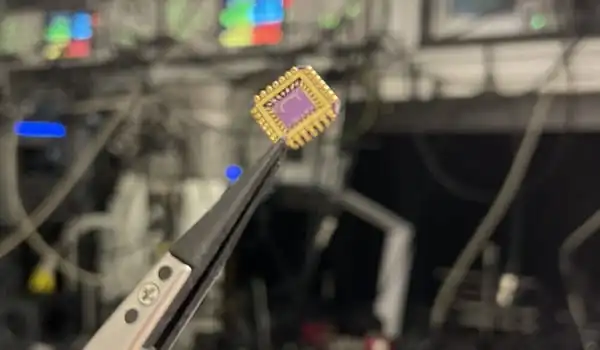
Quantum geometry and Valley selectivity
There are two pockets of electron valleys (K and K’) in bilayer graphene: when an electric field is applied perpendicularly, the quantum geometrical properties of electrons in these two valleys can cause them to bend in opposite directions. As a result, their Hall effects are neutralized.
The researchers discovered that by shining circular polarized infrared light onto a bilayer graphene device, they were able to selectively excite one specific valley population of electrons in the material, resulting in photovoltage perpendicular to the normal electron flow. As Koppens points out, “We now engineered and configured the device so that current only flows with light illumination. We were able to avoid the background noise that interferes with measurements and achieve detection sensitivity several orders of magnitude higher than any other 2D material.” This advancement is significant because conventional photodetectors frequently require large voltage biases, which can result in “dark currents” that flow even when no light is present.
“We can control the bending of the electrons with the out-of-plane electric field we apply,” Yin says. The Hall conductivity measures how much we can change the bending angle of these electrons. The Berry curvature [a property of quantum geometry] can be tuned by adjusting the voltage ‘knob,’ leading to massive Hall conductivity.”
As Koppens concludes, the study’s findings open up a new world of detection and imaging applications. ” Because bilayer graphene can be transformed from semimetal to semiconductor with a very small bandgap, it can detect photons with very small energies, this discovery could have significant implications in applications for infrared and terahertz sensing.
The possibilities are endless, and future research on new 2D materials, such as the moiré material twisted bilayer graphene, may uncover novel methods of controlling electron flows and unconventional opto-electronic properties.