Scientists Jim Watson and Alexey Olovnikov independently discovered a flaw in the way human DNA is duplicated half a century ago. The end-replication problem is a quirk of linear DNA replication that caused telomeres that protect the ends of chromosomes to get shorter with each round of replication.
Liz Blackburn and Carol Greider discovered telomerase, an enzyme that adds telomeric repeats to the ends of chromosomes. Titia de Lange of Rockefeller adds, “Everyone thought the case was closed.”
Now, new research published in Nature suggests that there are two end-replication problems, not one. Further, telomerase is only part of the solution – cells also use the CST-Polα-primase complex, which has been extensively studied in de Lange’s laboratory. “For many decades we thought we knew what the end-replication problem was and how it was solved by telomerase,” says de Lange. “It turns out we had missed half the problem.”
The DNA replication machinery cannot not fully duplicate the end of a linear DNA, much the same way that you can’t paint the floor under your feet.
Hiro Takai
The leading-strand problem
Since the description of the DNA double helix, it has been known that DNA comprises two complimentary strands that run in opposite directions: one from 5′ to 3′ and the other from 3′ to 5′. During DNA replication, the replication machinery, also known as the replisome, separates the two strands. Leading-strand synthesis refers to the method by which the replisome copies the 3′ to 5′ strand uninterrupted. However, the other strand is constructed in small retrograde steps from several fragments (Okazaki fragments) that are eventually sewn together.
The technique is quite straightforward till the chromosome terminates. When replicating the telomere, leading-strand DNA replication should duplicate the CCCTAA repeats to create the TTAGGG repeat strand, while lagging-strand synthesis should do the opposite, creating new CCCTAA repeats. The end-replication problem emerges when leading strand synthesis fails to replicate the last segment of the telomere, resulting in a blunt leading-end telomere lacking the characteristic and critical 3′ overhang. Telomerase addresses this issue by introducing single-stranded TTAGGG repeats at the telomere end. In terms of the lagging strand, DNA synthesis should go smoothly. It could begin the final Okazaki fragment somewhere along the 3′ overhang.
“The DNA replication machinery cannot not fully duplicate the end of a linear DNA, much the same way that you can’t paint the floor under your feet” says Hiro Takai, senior staff scientist in the de Lange lab and lead author on the paper.
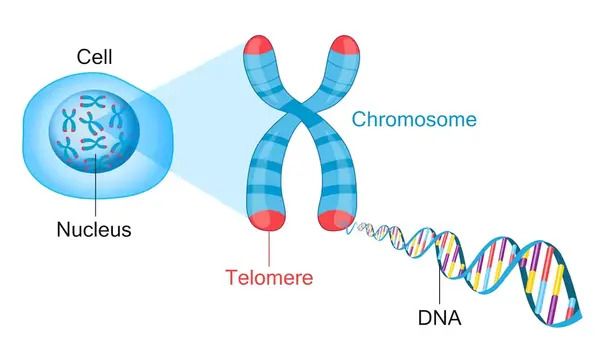
The lagging-strand problem
As descriptions of biological processes go, this model looked watertight. Until Takai made a surprising discovery while working on cells that lacked molecular machinery called the CST-Polα-primase complex. He and others had previously shown that CST-Polα-primase can replenish CCCTAA repeats at telomeres that had been attacked by DNA-degrading enzymes known as nucleases. This new data revealed something unexpected: not only was the leading strand in need of help — he found evidence that the end of the lagging strand could also not be synthesized by the replisome.
Takai’s work suggested that the end-replication problem was twice as serious as previously thought, impacting both strands of DNA. “The results just didn’t fit with the model for telomere replication,” de Lange says. “At that point, Hiro and I realized that either his results were not right or the model was wrong. As his results seemed very solid to me, we needed to revisit the model.”
De Lange contacted Joseph T. P. Yeeles, a biochemist who studies DNA replication at the Laboratory of Molecular Biology in Cambridge (the same lab where Watson and Crick worked on the structure of the DNA double helix). Yeeles agreed that it would be good to take a close look at how the replisome behaves at the end of a linear DNA template. Could the replisome use a 3′ overhang to make the last Okazaki fragment, as was proposed?
Yeeles’ in vitro replication experiments yielded unambiguous results. The replisome does not produce Okazaki fragments on the 3′ overhang; instead, it ends lagging-strand synthesis long before the leading strand reaches the 5′ end. This second end-replication issue implies that with each division, both strands of DNA shrink. Hiro’s findings suggests that CST-Polα-primase solved the second end-replication problem, that of the lagging strand, while telomerase only prevented this at the leading strand.
Takai spent the next four years developing new assays to validate Yeeles’ findings in vivo. He measured the amount of DNA lost owing to lagging-strand end-replication, revealing how many CCCAAT repeats required to be inserted by CST-Polα-primase to maintain telomeres intact.
The findings challenge our knowledge of telomere biology, necessitating textbook revisions. However, the findings may have clinical consequences. Mutations in CST-Polα-primase can cause telomere problems, including Coats plus syndrome, which causes defects in the eye, brain, bones, and GI system. Strides in tackling these severe conditions may be made possible by gaining a deeper knowledge of how we preserve our telomeres.