In recent years, the development of implantable devices for diabetes management has been a promising field of study and innovation. These devices are designed to make blood glucose control more convenient and effective, decreasing or eliminating the need for traditional insulin injections.
Engineers created an implanted device with hundreds of thousands of islet cells and its own onboard oxygen factory to keep the cells healthy. A device like this could help Type 1 diabetes patients avoid the need for insulin injections.
Implanting pancreatic islet cells that can manufacture insulin when needed is one potential technique for treating Type 1 diabetes, which could liberate patients from needing regular insulin injections. However, once the cells are transplanted, they eventually run out of oxygen and cease generating insulin.
To overcome this barrier, MIT researchers created a unique implanted device that not only contains hundreds of thousands of insulin-producing islet cells but also has its own on-board oxygen factory that manufactures oxygen by splitting water vapor found in the body.
When implanted into diabetic mice, the researchers demonstrated that this device could maintain the mice’s blood glucose levels steady for at least a month. The researchers are now hoping to develop a larger version of the device, around the size of a stick of chewing gum, that will be tested in persons with Type 1 diabetes.
You can think of this as a living medical device that is made from human cells that secrete insulin, along with an electronic life support system. We’re excited by the progress so far, and we really are optimistic that this technology could end up helping patients.
Daniel Anderson
“You can think of this as a living medical device that is made from human cells that secrete insulin, along with an electronic life support-system. We’re excited by the progress so far, and we really are optimistic that this technology could end up helping patients,” says Daniel Anderson, a professor in MIT’s Department of Chemical Engineering, a member of MIT’s Koch Institute for Integrative Cancer Research and Institute for Medical Engineering and Science (IMES), and the senior author of the study.
While the researchers’ main focus is on diabetes treatment, they say that this kind of device could also be adapted to treat other diseases that require repeated delivery of therapeutic proteins.
MIT Research Scientist Siddharth Krishnan is the lead author of the paper, which appears today in the Proceedings of the National Academy of Sciences. The research team also includes several other researchers from MIT, including Robert Langer, the David H. Koch Institute Professor at MIT and a member of the Koch Institute, as well as researchers from Boston Children’s Hospital.
Replacing injections
Most Type 1 diabetes patients must carefully check their blood glucose levels and inject themselves with insulin at least once each day. However, this procedure does not replicate the body’s natural ability to regulate blood glucose levels.
“The vast majority of insulin-dependent diabetics are injecting themselves with insulin and doing their best, but they do not have healthy blood sugar levels,” Anderson explains. “When you look at their blood sugar levels, even people who are very careful, they just can’t match what a living pancreas can do.”
A better alternative would be to transplant cells that produce insulin whenever they detect surges in the patient’s blood glucose levels. Some diabetes patients have received transplanted islet cells from human cadavers, which can achieve long-term control of diabetes; however, these patients have to take immunosuppressive drugs to prevent their body from rejecting the implanted cells.
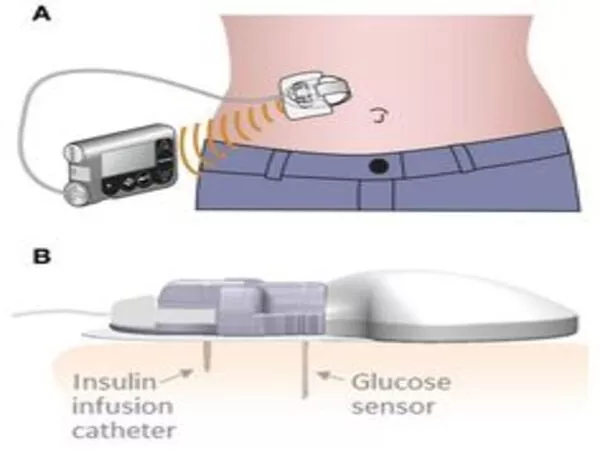
Researchers have now demonstrated similar effectiveness with islet cells produced from stem cells, but patients who receive those cells must also take immunosuppressive medicines. Another option for avoiding the use of immunosuppressive medicines is to encapsulate the transplanted cells within a flexible device that protects the cells from the immune system. However, finding a consistent oxygen source for these enclosed cells has been difficult.
Some experimental devices, including one evaluated in clinical trials, have an oxygen chamber that can supply the cells, but this chamber must be reloaded on a regular basis. Other researchers have created implants that contain chemical compounds that can manufacture oxygen, but these too eventually run out.
The MIT scientists explored a different strategy, splitting water to potentially generate oxygen indefinitely. This is accomplished by the use of a proton-exchange membrane, which is housed within the device and was initially used to generate hydrogen in fuel cells. This membrane has the ability to split water vapor (which is common in the body) into hydrogen, which diffuses harmlessly away, and oxygen, which goes into a storage chamber and feeds the islet cells via a thin, oxygen-permeable membrane.
This method has the enormous advantage of not requiring any wires or batteries. To split this water vapor, a little voltage (approximately 2 volts) is required, which is generated by a phenomenon known as resonant inductive coupling. A tuned magnetic coil located outside the body transmits power to a small, flexible antenna within the device, allowing for wireless power transfer. It does require an external coil, which the researchers anticipate could be worn as a patch on the patient’s skin.
Drugs on demand
The researchers tested their device, which is roughly the size of a quarter, in diabetic mice after building it. The device with the oxygen-generating, water-splitting membrane was given to one set of mice, whereas the other received islet cells without any supplemental oxygen. In mice with completely working immune systems, the devices were implanted just beneath the skin.
The researchers discovered that mice implanted with the oxygen-generating gadget could keep their blood glucose levels normal, comparable to healthy animals. However, mice with the nonoxygenated device were hyperglycemic (had high blood sugar) within two weeks.
When any type of medical device is implanted in the body, the immune system’s attack causes a buildup of scar tissue termed fibrosis, which can limit the device’s function. Although scar tissue did form around the implants utilized in this trial, the device’s success in managing blood glucose levels implies that insulin and glucose were still able to diffuse out of the device.
This method could potentially be used to transfer cells that create other types of therapeutic proteins that must be administered over time. The researchers demonstrated that the gadget could also retain alive cells that make erythropoietin, a protein that increases red blood cell synthesis, in this study.
“We’re optimistic that it will be possible to make living medical devices that can reside in the body and produce drugs as needed,” Anderson says. “There are a variety of diseases where patients need to take proteins exogenously, sometimes very frequently. If we can replace the need for infusions every other week with a single implant that can act for a long time, I think that could really help a lot of patients.”
The researchers intend to modify the device for use in larger animals and, eventually, humans. They intend to build an implant around the size of a chewing gum stick for human use. They also intend to see if the device can stay in the body for extended periods of time.
“The materials we’ve used are inherently stable and long-lived, so I think that kind of long-term operation is within the realm of possibility, and that’s what we’re working on,” Krishnan adds.
“We are very excited about these findings, which we believe could someday provide a whole new way of treating diabetes and possibly other diseases,” Langer says.