Kagome metals are a class of materials that have a unique crystal structure consisting of interconnected triangles arranged in a hexagonal lattice. These materials can exhibit superconductivity, a phenomenon in which they can conduct electricity with zero resistance at low temperatures.
A distinct disorder-driven superconductor-insulator transition was discovered in a recent study. This first electric control of superconductivity and the quantum Hall effect in a candidate material for future low-energy electronics holds the promise of lowering computing’s rising, unsustainable energy cost.
A new international collaboration led by RMIT published in February discovered a distinct disorder-driven bosonic superconductor-insulator transition for the first time. The discovery paints a global picture of the giant anomalous Hall effect and reveals its relationship with the unusual charge density wave in the AV3Sb5 kagome metal family, which could be used in future ultra-low energy electronics.
Superconductors, which can transmit electricity without losing energy, hold great promise for the development of future low-energy electronics technologies and are already used in a variety of applications such as hover trains and high-strength magnets (such as medical MRIs). However, the precise mechanism by which superconductivity forms and operates in many materials is still unknown, limiting its applications.
The results of the gated AHE also revealed that the most possible origin of the AHE is skew scattering and this further improves our understanding on the kagome metal. But we have not yet observed superconductor-insulator transition in 40 nm nanoflakes. We must further try thinner CsV3Sb5 nanoflakes to explore this.
Dr. Guolin Zheng
A new kagome superconductor family, AV3Sb5, has recently sparked intense interest due to its novel properties. Materials with the name ‘Kagome’ feature an unusual lattice pattern named after a Japanese basket-weave pattern with corner-sharing triangles.
The AV3Sb5 materials (where A is caesium, rubidium, or potassium) provide ideal platforms for physics studies such as topology and strong correlations, but the origin of the material’s giant anomalous Hall effect and superconductivity remains unknown despite numerous recent investigations.
The FLEET-led collaboration of RMIT University (Australia) and the High Magnetic Field Laboratory (China) confirms for the first time the electric control of superconductivity and AHE in a van der Waals kagome metal CsV3Sb5.
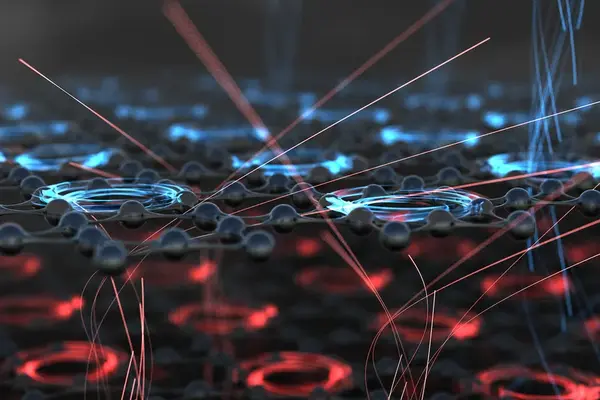
Manipulating giant anomalous Hall effect via reversible proton intercalation
Possessing topological electron bands and geometrical frustration of vanadium lattices, the layered kagome metals AV3Sb5 have attracted great interests in condensed matter physics due to the many quantum phenomena that they support including:
- unconventional, novel nematic order
- chiral charge density order
- giant anomalous Hall effect (AHE), and
- the interplay between two-gap superconductivity and charge density wave (CDW) in AV3Sb5.
Furthermore, despite several recently proposed mechanisms, such as extrinsic skew scattering of Dirac quasiparticles with frustrated magnetic sublattice, orbital currents of novel chiral charge order, and the chiral flux phase in the CDW phase, the origin of giant AHE in AV3Sb5 and its correlation with chiral CDW remain elusive.
“We had previously obtained a number of intriguing results using the proton gate technique in vdW spintronic devices. We would like to apply this technique to AV3Sb5, which has a similar carrier density level, because it can effectively modulate the carrier density up to 1021 cm-3 “FLEET Research Fellow Dr Guolin Zheng, the study’s first author, says (RMIT).
“The ability to tune the carrier density and the corresponding Fermi surfaces would be critical in understanding and manipulating these novel quantum states, potentially resulting in some exotic quantum phase transitions.”
The researchers chose to test this theory on CsV3Sb5, which has the most spare atom space for proton intercalation. Based on the team’s extensive experience in this field, the devices were easily designed and manufactured. Their subsequent results with CsV3Sb5 were highly dependent on material thickness.
“It was very difficult to effectively modulate the ‘thicker’ nanoflakes (more than 100 nm),” says co-first author, FLEET Research Fellow Dr Cheng Tan (RMIT). But when the thickness went down to around 40 nm, the injection of the proton became quite easy,” says Cheng. “We even found that the injection is highly reversible. Indeed, we have seldom met such a proton-friendly material!”
Interestingly, with evolving proton intercalation, the carrier type (or the’sign’ of the Hall effect) could be modulated to either hole or electron type, and the amplitude of the AHEs obtained could also be effectively tuned. Further experimental and theoretical research indicates that the Fermi level shift in the reconstructed band structures is responsible for the dramatic modulation of giant AHE.
“The results of the gated AHE also revealed that the most possible origin of the AHE is skew scattering and this further improves our understanding on the kagome metal,” explains Guolin. “But we have not yet observed superconductor-insulator transition in 40 nm nanoflakes. We must further try thinner CsV3Sb5 nanoflakes to explore this.”
Proton intercalation induced superconductor-to-‘failed insulator’ transition
The unusual coexistence of electronic correlations and band topology in AV3Sb5 allows for the investigation of intriguing transitions of these correlated states, such as the superconductor-insulator transition, a quantum phase transition that is typically tuned by disorders, magnetic fields, and electric gating. The team went a step further in exploring the potential quantum phase transitions in CsV3Sb5 by reducing the number of atomic layers.
“At first, I tried some 10 nm ultrathin nanoflakes,” Cheng says. “I did notice that the critical temperatures of the superconductivity phase decreased as proton intercalation increased, but I couldn’t definitively say that superconductivity vanished because it could still exist at milliKelvin temperatures that we couldn’t reach. When I tried to increase the proton intercalation further, the devices became very fragile.”
So Cheng changed his strategy and dealt with the 1020nm thicker nanoflakes while also experimenting with different electrode materials in search of a better electrical contact. This strategy was a success. Surprisingly, the team discovered that the critical temperature of the CDW phase decreased and that the temperature-dependent resistance curves show a clear superconductor-to-insulator transition as proton injection increased.
“The disorder was introduced by proton intercalation, which suppressed both CDW and superconducting phase coherence,” says co-author A/Prof Lan Wang (also at RMIT). “This gave rise to a ‘failed insulator,’ a superconductor-insulator transition associated with localized Cooper pairs and featuring a saturated sheet resistance reaching up to 106? for temperatures approaching zero.”
“Our findings reveal a distinct disorder-driven bosonic superconductor-insulator transition, a global picture of the giant AHE, and a link between it and the unusual CDW in the AV3Sb5 family. This significant and electrically controlled superconductor-insulator transition and anomalous Hall effect in kagome metals should pique the interest of more researchers interested in the fascinating physics, which holds promise for energy-saving nanoelectronic devices.”