The scientists reproduced a ‘quantum bomb tester’ in a classical droplet test in a work that could help bridge certain gaps in quantum theory.
What you see is what you get in our everyday classical world. A ball is merely a ball, and when thrown through the air, its trajectory is simple and predictable. However, if that ball was compressed to the size of an atom or smaller, its behavior would change to a quantum, fuzzy reality. The ball would exist as a wave of conceivable particle states as well as a physical particle. And this wave-particle duality can result in some strange and devious events.
One of the more unusual possibilities stems from a thought experiment known as the “quantum bomb tester.” According to the experiment, a quantum particle, such as a photon, could operate as a telekinetic bomb detector. The photon, as both a particle and a wave, might theoretically perceive the presence of a bomb without physically interacting with it.
The concept is mathematically sound and consistent with what the equations controlling quantum mechanics allow. But when it comes to explaining how a particle could perform such a bomb-sniffing feat, scientists are at a loss. The problem is that quantum particles are intrinsically shifty, in-between, and undefinable. In other words, scientists must just believe that it works.
But mathematicians at MIT are hoping to dispel some of the mystery and ultimately establish a more concrete picture of quantum mechanics. They have now shown that they can recreate an analog of the quantum bomb tester and generate the behavior that the experiment predicts. They’ve done so not in an exotic, microscopic, quantum setting, but in a seemingly mundane, classical, tabletop setup.
Here we have a classical system that gives the same statistics as arises in the quantum bomb test, which is considered one of the wonders of the quantum world. In fact, we discover that the phenomenon isn’t all that wonderful after all. And there is just another example of quantum activity that can be explained by a local realist.
John Bush
The team reports reproducing the quantum bomb tester in an experiment involving bouncing droplets in a publication published today in Physical Review A. The researchers discovered that the droplet’s interaction with its own waves is analogous to the quantum wave-particle behavior of a photon: When dropped into a configuration identical to that proposed in the quantum bomb test, the droplet performs exactly as predicted for the photon in terms of statistics. If there were a bomb in the setup 50% of the time, the droplet, like the photon, would detect it 25% of the time without physically engaging with it.
The fact that the statistics in both experiments agree suggests that something in the droplet’s classical dynamics could be at the root of a photon’s otherwise enigmatic quantum behavior. The study, according to the researchers, is another link between two realities: the observable, classical world and the fuzzier quantum one.
“Here we have a classical system that gives the same statistics as arises in the quantum bomb test, which is considered one of the wonders of the quantum world,” said study author John Bush, an applied mathematics professor at MIT. “In fact, we discover that the phenomenon isn’t all that wonderful after all. And there is just another example of quantum activity that can be explained by a local realist.”
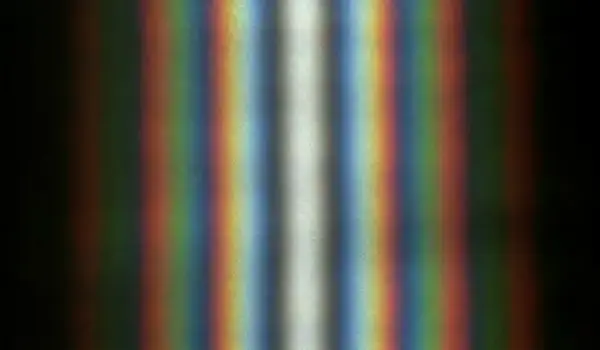
Making waves
To some physicists, quantum mechanics leaves too much to the imagination and doesn’t say enough about the actual dynamics from which such weird phenomena supposedly arise. In 1927, in an attempt to crystallize quantum mechanics, physicist Louis de Broglie presented the pilot wave theory – a still-controversial idea that poses a particle’s quantum behavior is determined not by an intangible, statistical wave of possible states but by a physical “pilot” wave of its own making, that guides the particle through space.
Until 2005, when physicist Yves Couder demonstrated that de Broglie’s quantum waves could be recreated and investigated in a classical, fluid-based experiment, the hypothesis was widely dismissed. The setup consists of a bath of fluid that is manipulated to vibrate up and down subtly, but not sufficiently to generate waves on its own. A millimeter-sized droplet of the same fluid is then dispersed over the bath, and when it bounces off the surface, it resonates with the vibrations of the bath, creating what physicists call a standing wave field, which “pilots,” or pushes, the droplet along. The effect is that a droplet appears to move along a rippled surface in patterns that match de Broglie’s pilot wave hypothesis.
For the last 13 years, Bush has worked to refine and extend Couder’s hydrodynamic pilot wave experiments and has successfully used the setup to observe droplets exhibiting emergent, quantum-like behavior, including quantum tunneling, single-particle diffraction, and surreal trajectories.
“It turns out that this hydrodynamic pilot-wave experiment exhibits many features of quantum systems which were previously thought to be impossible to understand from a classical perspective,” Bush says.
Bombs away
In their new study, he and Frumkin took on the quantum bomb tester. The thought experiment begins with a conceptual interferometer – essentially, two corridors of the same length that branch out from the same starting point, then turn and converge, forming a rhombus-like configuration as the corridors continue on, each ending in a respective detector.
According to quantum mechanics, if a photon is fired from the interferometer’s starting point, through a beamsplitter, the particle should travel down one of the two corridors with equal probability. Meanwhile, the photon’s mysterious “wave function,” or the sum of all its possible states, travels down both corridors simultaneously. The wave function interferes in such a way to ensure that the particle only appears at one detector (let’s call this D1) and never the other (D2). Hence, the photon should be detected at D1 100 percent of the time, regardless of which corridor it traveled through.
If there is a bomb in one of the two corridors and a photon travels down it, the bomb is triggered, the setup is blown up, and no photon is registered at either detector. But if the photon proceeds down the corridor without the bomb, something strange happens: its wave function is cut short in one of the corridors by the bomb. The wave does not set off the bomb since it is not nearly a particle. However, the wave interference is changed so that the particle is recognized with equal probability at D1 and D2. Any signal at D2 therefore would mean that a photon has detected the presence of the bomb, without physically interacting with it. If the bomb is present 50 percent of the time, then this weird quantum bomb detection should occur 25 percent of the time.
In their new study, Bush and Frumkin set up an analogous experiment to see if this quantum behavior could emerge in classical droplets. Into a bath of silicon oil, they submerged a structure similar to the rhombus-like corridors in the thought experiment. They then carefully dispensed tiny oil droplets into the bath and tracked their paths. They added a structure to one side of the rhombus to mimic a bomb-like object and observed how the droplet and its wave patterns changed in response.
Finally, they discovered that 25% of the time, a droplet bounced around the corridor without the “bomb,” while its pilot waves interacted with the bomb structure, pushing the droplet away from the bomb. From this vantage point, the droplet was able to “sense” the bomb-like object without coming into actual touch with it. While the droplet displayed quantum-like behavior, the scientists could clearly see that this behavior came from the droplet’s waves, which physically kept the droplet away from the bomb. According to the researchers, these dynamics may also help to explain the strange behavior of quantum particles.