DNA damage can be repaired through a variety of mechanisms, including antioxidant enzyme action. Antioxidant enzymes play an important role in DNA integrity by removing or repairing damaged DNA bases. In times of crisis, the nucleus summons antioxidant enzymes to its aid. The fact that the nucleus is metabolically active represents a significant paradigm shift with implications for cancer research.
Summary points
- According to the findings of a new study published in Molecular Systems Biology by researchers at the CRG in Barcelona and CeMM/Medical University of Vienna, the human nucleus is metabolically active.
- In times of crisis, such as widespread DNA damage, the nucleus defends itself by repurposing mitochondrial machinery to perform urgent repairs that jeopardize the genome’s integrity.
- The findings represent a paradigm shift because the nucleus was previously thought to be metabolically inactive, importing all of its needs via supply chains in the cytoplasm.
- Cancer hijacks cellular metabolism to allow for unrestricted growth. The findings can help direct future cancer research by providing new clues to overcome drug resistance and, eventually, the development of new treatments.
Main text
A typical human cell is metabolically active, rife with chemical reactions that convert nutrients into energy and useful products that keep life going. These reactions also produce reactive oxygen species, which are hazardous byproducts such as hydrogen peroxide, which damage the building blocks of DNA in the same way that oxygen and water corrode metal and form rust. Just as rust causes buildings to collapse, reactive oxygen species endangers the integrity of a genome.
Cells are thought to delicately balance their energy needs while avoiding DNA damage by containing metabolic activity outside the nucleus, in the cytoplasm, and in the mitochondria. Antioxidant enzymes are used to mop up reactive oxygen species before they reach DNA, a defensive strategy that protects the approximately 3 billion nucleotides from potentially catastrophic mutations. If DNA damage occurs, cells pause for a brief moment to repair themselves, synthesising new building blocks and filling in the gaps.
Where there’s smoke there’s fire, and where there’s reactive oxygen species there are metabolic enzymes at work. Historically, we’ve thought of the nucleus as a metabolically inert organelle that imports all its needs from the cytoplasm, but our study demonstrates that another type of metabolism exists in cells and is found in the nucleus.
Dr. Sara Sdelci
Despite the importance of cellular metabolism in genome integrity, no systematic, unbiased research has been conducted on how metabolic perturbations affect the DNA damage and repair process. This is especially important for diseases like cancer, which have the ability to hijack metabolic processes for unrestricted growth.
A research team led by Sara Sdelci at the Centre for Genomic Regulation (CRG) in Barcelona and Joanna Loizou at the Austrian Academy of Sciences’ CeMM Research Center for Molecular Medicine in Vienna and the Medical University of Vienna took on this challenge by conducting a series of experiments to determine which metabolic enzymes and processes are required for a cell’s DNA damage response. The research was published in the journal Molecular Systems Biology today.
The researchers used etoposide, a common chemotherapy medication, to induce DNA damage in human cell lines. Etoposide works by breaking down DNA strands and inhibiting an enzyme that aids in DNA repair. Surprisingly, inducing DNA damage resulted in the generation of reactive oxygen species, which accumulated inside the nucleus. In response to DNA damage, the researchers discovered that cellular respiratory enzymes, a major source of reactive oxygen species, relocated from the mitochondria to the nucleus.
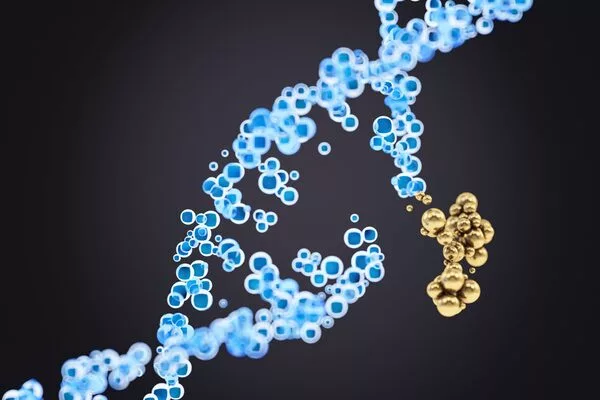
The findings represent a paradigm shift in cellular biology because it suggests the nucleus is metabolically active. “Where there’s smoke there’s fire, and where there’s reactive oxygen species there are metabolic enzymes at work. Historically, we’ve thought of the nucleus as a metabolically inert organelle that imports all its needs from the cytoplasm, but our study demonstrates that another type of metabolism exists in cells and is found in the nucleus,” says Dr. Sara Sdelci, corresponding author of the study and Group Leader at the Centre for Genomic Regulation.
In addition, the researchers used CRISPR-Cas9 to identify all of the metabolic genes required for cell survival in this scenario. These experiments revealed that cells direct the antioxidant enzyme PRDX1, which is normally found in mitochondria, to travel to the nucleus and scavenge reactive oxygen species to prevent further damage. PRDX1 was also discovered to repair the damage by regulating the cellular availability of aspartate, a raw material required for the synthesis of nucleotides, the DNA building blocks.
“PRDX1 is similar to a robotic pool cleaner.” It is known that cells use it to keep their interiors ‘clean’ and prevent the accumulation of reactive oxygen species, but it has never been used at the nuclear level. “This is evidence that, in a crisis, the nucleus responds by appropriating mitochondrial machinery and establishing an emergency rapid-industrialisation policy,” Dr. Sdelci says.
The findings may help to direct future cancer research. Some anti-cancer drugs, such as etoposide, kill tumor cells by damaging their DNA and interfering with the repair process. If enough damage accumulates, the cancer cell initiates an autodestructive process.
During their experiments, the researchers discovered that knocking out metabolic genes important for cellular respiration (the process by which energy is generated from oxygen and nutrients) made normal healthy cells resistant to etoposide. The discovery is significant because many cancer cells are glycolytic, which means they generate energy even in the presence of oxygen without engaging in cellular respiration. As a result, etoposide and other chemotherapies with a similar mechanism are likely to be ineffective in treating glycolytic tumors.
The study’s authors advocate for the development of new strategies, such as dual treatment combining etoposide with drugs that also increase the generation of reactive oxygen species, in order to overcome drug resistance and kill cancer cells more quickly. They also hypothesize that combining etoposide with inhibitors of nucleotide synthesis processes could enhance the drug’s effect by preventing DNA repair and ensuring cancer cells self-destruct properly.
Dr. Joanna Loizou, Group Leader at the Centre for Molecular Medicine and the Medical University of Vienna, emphasizes the importance of using data-driven approaches to discover new biological processes. ‘We learned about how the two fundamental cellular processes of DNA repair and metabolism are intertwined by using unbiased technologies like CRISPR-Cas9 screening and metabolomics. Our findings shed light on how targeting these two pathways in cancer could improve therapeutic outcomes for patients’.