What causes galaxies to form, and what holds them together? Astronomers believe that dark matter is important. However, it has not yet been possible to directly prove the existence of dark matter. A research team led by scientists from the Technical University of Munich (TUM) has measured for the first time the survival rate of antihelium nuclei from the depths of the galaxy – a prerequisite for the indirect search for Dark Matter.
There are numerous indications that dark matter exists. Calculations based on how galaxies move in galactic clusters or how fast stars circle the center of a galaxy indicate that there must be far more mass present than what we can see. Approximately 85 percent of our Milky Way for example consists of a substance that is not visible and which can only be detected based on its gravitational effects. As of today, it has still not been possible to directly prove the existence of this material.
Several theoretical models of dark matter predict that it could be composed of particles that interact weakly with one another. This produces antihelium-3 nuclei, which consist of two antiprotons and one antineutron. These nuclei are also generated in high-energy collisions between cosmic radiation and common matter like hydrogen and helium – however, with energies different from those that would be expected in the interaction of dark matter particles.
This is an excellent example of an interdisciplinary analysis that illustrates how measurements at particle accelerators can be directly linked with the study of cosmic rays in space.
Laura Fabbietti
In both processes, the antiparticles originate in the depths of the galaxy, several tens of thousands of lightyears away from us. After their creation, a part of them makes its way in our direction. How many of these particles survive this journey unscathed and reach the vicinity of the Earth as messengers of their formation process determines the transparency of the Milky Way for antihelium nuclei. Until now scientists have only been able to roughly estimate this value. However, an improved approximation of transparency, a unit of measure for the number and energies of antinuclei, will be important for interpreting future antihelium measurements.
LHC particle accelerator as antimatter factory
Researchers from the ALICE collaboration have now carried out measurements that have enabled them to determine transparency more precisely for the first time. ALICE stands for A Large Ion Collider Experiment and is one of the largest experiments in the world to explore physics on the smallest length scales. ALICE is part of the Large Hadron Collider (LHC) at CERN.
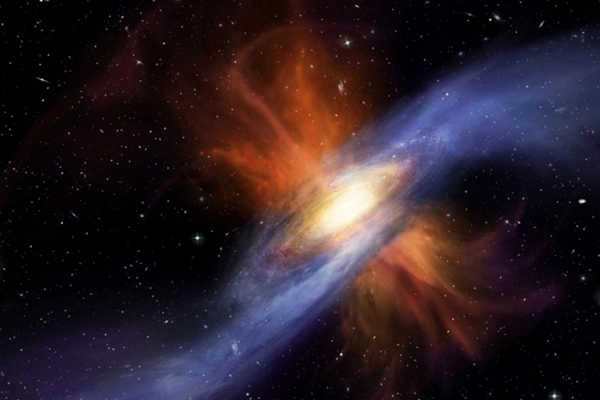
The LHC can generate large amounts of light antinuclei such as antihelium. To do so, protons and lead atoms are each put on a collision course. The collisions produce particle showers which are then recorded by the detector of the ALICE experiment. Thanks to several subsystems of the detector, the researchers can then detect the antihelium-3 nuclei that have formed and follow their trails in the detector material. This makes it possible to quantify the probability that an antihelium-3 nucleus will interact with the detector material and disappear. Scientists from TUM and the Excellence Cluster ORIGINS have contributed significantly to the analysis of the experimental data.
Galaxy transparent for antinuclei
Using simulations, the researchers were able to transfer the findings from the ALICE experiment to the entire galaxy. The result: About half of the antihelium-3 nuclei which were expected to be generated in the interaction of dark matter particles would reach the vicinity of the Earth. Our Milky Way is thus 50 percent permeable for these antinuclei. For antinuclei generated in collisions between cosmic radiation and the interstellar medium, the resulting transparency varies from 25 to 90 percent with increasing antihelium-3 momentum. However, these antinuclei can be distinguished from those generated from dark matter based on their higher energy.
This means that antihelium nuclei can not only travel long distances in the Milky Way, but also serve as important informants in future experiments: Depending on how many antinuclei arrive at the Earth and with which energies, the origin of these well-traveled messengers can be interpreted as cosmic rays or dark matter thanks to the new calculations.
Reference for future antinuclei measurements in space
“This is an excellent example of an interdisciplinary analysis that illustrates how measurements at particle accelerators can be directly linked with the study of cosmic rays in space,” says ORIGINS scientist Prof. Laura Fabbietti of the TUM School of Natural Sciences. The results from the ALICE experiment at the LHC are of great importance for the search for antimatter in space with the AMS-02 module (Alpha Magnetic Spectrometer) on the International Space Station (ISS). Starting in 2025 the GAPS balloon experiment over the Arctic will also examine incoming cosmic rays for antihelium-3.
Antihelium nuclei as messengers from the depths of the galaxy @TU_Muenchen @NaturePhysics https://t.co/l1K0atDXF4
— Phys.org (@physorg_com) December 12, 2022