According to a Penn State and Brown University research team, rocks buried deep in past subduction zones (where tectonic plates contact) could help scientists make better predictions about how these zones behave in the years between major earthquakes.
Clues from rock formations in Alaska and Japan helped scientists construct a novel model for predicting pressure solution activity in subduction zones, according to a study published in the journal Science Advances. Sedimentary rocks are made up of grains surrounded by pores that contain water. When rocks are pushed together under high pressure, the grains dissolve at their borders into the water present in the pores, resulting in a pressure solution. This permits the rocks to deform or change shape, impacting how tectonic plates travel past one another.
“It’s like when you go ice skating — the blade on the surface ends up melting the ice, which allows you to glide along,” said corresponding author Donald Fisher, professor of geosciences at Penn State. “In rocks, what happens is quartz grains dissolve at stressed contacts and the dissolved material moves to cracks where it precipitates.”
This work allows us to test a flow law, or model, that describes the rate of pressure solution in ancient rocks that were once down at the plate boundary and have been exhumed to the surface. And we can apply this to active margins that are moving today.
Donald Fisher
The world’s most powerful earthquakes occur in subduction zones, where one tectonic plate slides beneath another. When these plates become trapped together, stress builds up in the Earth’s crust, similar to how a rubber band is stretched. An earthquake occurs when enough tension accumulates to overcome the friction that holds the plates together, similar to a rubber band snapping.
“We’ve shown that pressure solution is a fundamental process during the interseismic period in subduction zones,” Fisher went on to say. “The occurrence of this pressure solution can really affect the amount of elastic strain that accumulates in different parts of the seismogenic zone.”
Pressure solution is difficult to explore in the laboratory because it typically occurs very slowly over thousands to millions of years, Fisher said. Speeding up the process in the lab requires higher temperatures, which produces other changes in rocks that impact the experiments.
The scientists instead turned to rocks that once experienced these tectonic pressures and were later brought to the surface by geological processes. The rocks show microscopic shears — or breaks caused by strain — that contain textures that provide evidence for pressure solution, the scientists said.
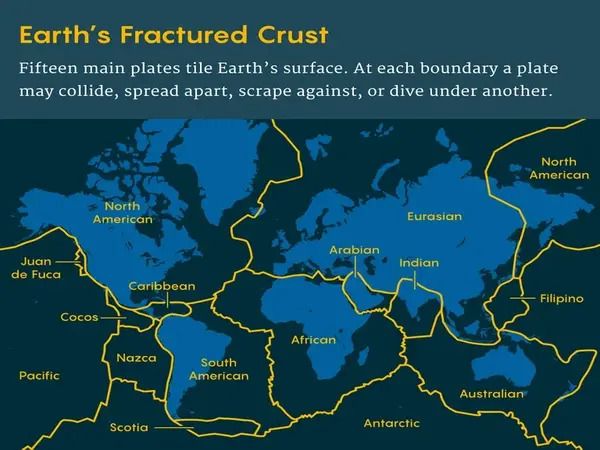
“This work allows us to test a flow law, or model, that describes the rate of pressure solution in ancient rocks that were once down at the plate boundary and have been exhumed to the surface,” Fisher said. “And we can apply this to active margins that are moving today.”
A previous study by another team of scientists linked stress the rocks experienced and strain rate — or how much they deformed. In the new work, Fisher and his colleague, Greg Hirth, professor at Brown University, created a more detailed model that considers factors like the rocks’ grain size and solubility, or how much of the rock material can dissolve into liquid.
“We were able to parameterize the solubility as a function of temperature and pressure, in a practical way that hadn’t been done before,” Fisher said. “So now we can plug in numbers — different grain sizes, different temperatures, different pressures and get the strain rate out of that.”
The results can help reveal where in the seismogenic layer — the range of depths at which most earthquakes occur — that strain is occurring.
The researchers applied their model to the Cascadia Subduction Zone, an active fault that stretches from northern California to Canada and passes through important towns such as Portland, Oregon, Seattle, and Vancouver, British Columbia.
The temperature and amount of strain built up at the plate boundary have been extensively researched, and the results of their model match crustal movements based on satellite observations, according to the experts.
“Cascadia is a great example because it’s late in the interseismic period — it’s been 300 years since the last major earthquake,” Fischer said. “We may experience one in our lifetime, which would be the biggest natural disaster that North America can anticipate in terms of the potential for shaking and resulting tsunami.”