A DESY-led research team used high-intensity X-rays to study a single catalyst nanoparticle in action. The experiment demonstrated for the first time how the chemical composition of a single nanoparticle’s surface changes under reaction conditions, making it more active. The findings of the team, led by DESY’s Andreas Stierle, have been published in the journal Science Advances. This research is an important step toward gaining a better understanding of real-world industrial catalytic materials.
Catalysts are substances that promote chemical reactions without being consumed. Catalysts are now used in a wide range of industrial processes, from fertilizer production to the manufacture of plastics. As a result, catalysts are extremely important economically. A well-known example is a catalytic converter found in automobile exhaust systems. These contain precious metals like platinum, rhodium, and palladium, which allow highly toxic carbon monoxide (CO) to be converted into carbon dioxide (CO2) while reducing the number of harmful nitrogen oxides (NOx).
“Despite their widespread use and importance, we still know very little about how the various catalysts work,” says Stierle, director of the DESY NanoLab. “That’s why we’ve long wanted to study real catalysts while they’re in use.” This is difficult because, in order to maximize the active surface, catalysts are typically used in the form of tiny nanoparticles, and the changes that affect their activity take place on their surface.
A research team has been using high-intensity X-rays to observe a single catalyst nanoparticle at work. The experiment has revealed for the first time how the chemical composition of the surface of an individual nanoparticle change under reaction conditions, making it more active.
Surface strain relates to the chemical composition
The DESY NanoLab team developed a technique for labeling individual nanoparticles and thus identifying them in a sample as part of the EU project Nanoscience Foundries and Fine Analysis (NFFA). “For the study, we grew nanoparticles of a platinum-rhodium alloy on a substrate in the lab and labeled one specific particle,” says co-author and DESY NanoLab project manager Thomas Keller. “The labeled particle has a diameter of around 100 nanometres and is similar to the particles used in a car’s catalytic converter.” A nanometre is one-millionth of a millimeter in length.
The team was able to create a detailed image of the nanoparticle using X-rays from the European Synchrotron Radiation Facility ESRF in Grenoble, France, as well as to measure the mechanical strain within its surface. “The surface strain is related to the surface composition, specifically the ratio of platinum to rhodium atoms,” says co-author Philipp Pleßow of the Karlsruhe Institute of Technology (KIT), whose group calculated strain as a function of surface composition. Conclusions about the chemical composition at the particle surface can be drawn by comparing observed and computed facet-dependent strain. The different surfaces of a nanoparticle are called facets, just like the facets of a cut gemstone.
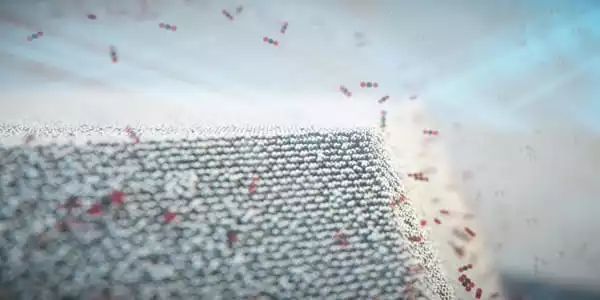
When the nanoparticle is formed, its surface is primarily composed of platinum atoms, as this configuration is energetically advantageous. The scientists, on the other hand, investigated the shape of the particle and its surface strain under various conditions, including the operating conditions of an automotive catalytic converter. They did this by heating the particle to around 430 degrees Celsius and passing carbon monoxide and oxygen molecules over it. “Under these reaction conditions, the rhodium inside the particle becomes mobile and migrates to the surface because it interacts with oxygen more strongly than the platinum,” Pleßow explains. Theory predicts this as well.
“As a result, the surface strain and particle shape change,” says DESY co-author Ivan Vartaniants, whose team converted the X-ray diffraction data into three-dimensional spatial images. “A facet-dependent rhodium enrichment occurs, resulting in the formation of additional corners and edges.” The chemical composition of the surface, as well as the shape and size of the particles, all have an impact on their function and efficiency. Scientists are only now beginning to understand how these are linked and how to control the structure and composition of the nanoparticles.
The X-rays allow researchers to detect changes of as little as 0.1 in a thousand in the strain, which in this experiment corresponds to a precision of about 0.0003 nanometres (0.3 picometres).
A crucial step towards analyzing industrial catalyst materials
“We can now observe the details of the structural changes in such catalyst nanoparticles while they are in operation for the first time,” says Stierle, Lead Scientist at DESY and professor of nanoscience at the University of Hamburg. “This is a significant step forward in understanding an entire class of reactions involving alloy nanoparticles.” Scientists at KIT and DESY want to investigate this more thoroughly through the new Collaborative Research Centre 1441, which is funded by the German Research Foundation (DFG) and titled “Tracking the Active Sites in Heterogeneous Catalysis for Emission Control (TrackAct).”
“Our research is an important step toward analyzing industrial catalytic materials,” says Stierle. Until now, scientists had to cultivate model systems in the laboratory in order to conduct such research. “We have reached the limit of what can be done in this study. We will be able to examine ten times smaller individual particles in real catalysts and under reaction conditions using DESY’s planned X-ray microscope PETRA IV.”