The search for superior semiconductors continues. A team of scientists at Columbia University led by Jack Tulyag, a Ph.D. student working with chemistry professor Milan Delor, report the fastest and most efficient semiconductor yet in Science: a superatomic substance called Re6Se8Cl2.
Semiconductors, most notably silicon, undergird the computers, telephones, and other electronic gadgets that power our daily lives, including the device on which you are reading this article. As widespread as semiconductors have grown, they have limitations. The atomic structure of any material vibrates, resulting in quantum particles known as phonons. Phonons, in turn, cause the particles that carry energy and information around electronic devices (electrons or electron-hole pairs termed excitons) to scatter in nanometers and femtoseconds. This means that energy is lost in the form of heat, and that information transfer has a speed limit.
Better options are being sought. A team of chemists at Columbia University led by Jack Tulyag, a PhD student working with chemistry professor Milan Delor, disclosed the quickest and most efficient semiconductor yet in Science: Re6Se8Cl2.
In terms of energy transport, Re6Se8Cl2 is the best semiconductor that we know of, at least so far. It was the opposite of what we expected. Instead of the slow movement we expected, we saw the fastest thing we’ve ever seen.
Milan Delor
Rather of scattering when they come into touch with phonons, excitons in Re6Se8Cl2 connect with them to form new quasiparticles known as acoustic exciton-polarons. Although polarons may be found in many materials, those in Re6Se8Cl2 have a unique property: they can travel in a ballistic, or scatter-free, manner. This ballistic tendency could lead to speedier and more efficient electronics in the future.
Acoustic exciton-polarons in Re6Se8Cl2 moved quickly in experiments, twice as fast as electrons in silicon, and spanned many microns of the sample in less than a nanosecond. Given that polarons have a lifetime of roughly 11 nanoseconds, the researchers believe the exciton-polarons may cover more than 25 micrometers at a time. Because these quasiparticles are controlled by light rather than an electrical current and gating, theoretical device processing speeds have the potential to approach femtoseconds – six orders of magnitude faster than existing Gigahertz circuits. Everything is at normal temperature.
“In terms of energy transport, Re6Se8Cl2 is the best semiconductor that we know of, at least so far,” Delor said in a statement.
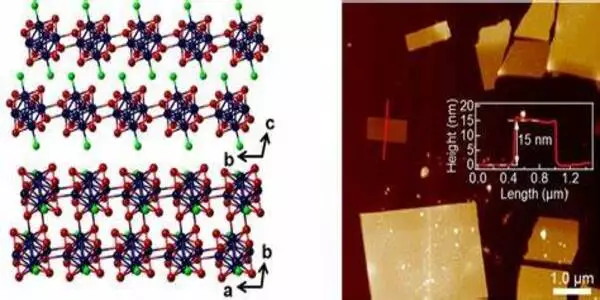
A Quantum Version of the Tortoise and the Hare
Re6Se8Cl2 is a superatomic semiconductor developed at collaborator Xavier Roy’s lab. Superatoms are clusters of atoms bonded together that behave like one large atom but have features different from the elements used to construct them. The Roy lab specializes in superatom synthesis, and they are a major focus of Columbia’s NSF-funded Material Research Science and Engineering Center on Precision Assembled Quantum Materials. Delor is interested in managing and manipulating energy transport via superatoms and other Columbia-developed materials. To accomplish this, the team creates super-resolution imaging technologies capable of capturing particles moving at ultrasmall and ultrafast scales.
When Tulyag first brought Re6Se8Cl2 into the lab, it wasn’t to search for a new and improved semiconductor – it was to test the resolution of the lab’s microscopes with a material that, in principle, shouldn’t have conducted much of anything. “It was the opposite of what we expected,” said Delor. “Instead of the slow movement we expected, we saw the fastest thing we’ve ever seen.”
Tulyag and his colleagues at the Delor group spent the next two years trying to figure out why Re6Se8Cl2 behaved so strangely, including developing an improved microscope with an extraordinary spatial and temporal resolution that can directly view polarons as they form and flow through the material. Petra Shih, a PhD student in Timothy Berkelbach’s lab, also devised a quantum mechanical model that explains the results.
The new quasiparticles are quick, but they achieve that speed by pacing themselves, similar to the parable of the tortoise and the hare, according to Delor. The fact that electrons can flow very quickly through silicon makes it a desired semiconductor, but like the proverbial hare, they bounce around too much and don’t actually get it very far, very fast in the end. Excitons in Re6Se8Cl2 are rather sluggish, but it is precisely because they are slow that they can meet and pair with similarly slow-moving acoustic phonons. The resulting quasiparticles are “heavy” and, like a tortoise, move slowly yet steadily. Acoustic exciton-polarons in Re6Se8Cl2 eventually move faster than electrons in silicon because they are not hampered by other phonons along the way.
The Semiconductor Search Continues
Re6Se8Cl2 can be peeled into atom-thin sheets, like many of the developing quantum materials being investigated at Columbia, which means it can possibly be coupled with other similar materials in the search for additional unique features. However, Re6Se8Cl2 is unlikely to ever be used in a commercial product because the first element in the molecule, Rhenium, is one of the rarest on the planet and thus exceedingly expensive.
But, with the Berkelbach group’s new theory in hand, as well as the advanced imaging technique developed by Tulyag and the Delor group to directly track the formation and movement of polarons, the team is ready to see if there are other superatomic contenders capable of breaking Re6Se8Cl2”s speed record.