Galaxies in the early universe were different from present-day galaxies. In the early universe, galaxies were smaller, more chaotic, and less well-formed than they are today. They were also undergoing a lot of merging and interaction, which helped to build up their size and structure over time. Additionally, the universe was much denser and hotter in the early stages, which affected the way that galaxies formed and evolved.
A new paper reports that the most sensitive telescope now searching for radio signals from cosmic dawn, an era around 200 million years after the Big Bang when stars ignited, has doubled its sensitivity. While they have yet to detect this radiation (the redshifted 21-centimeter line), they have set new limits on the elemental composition of galaxies during the Reionization Epoch. Early galaxies appear to be low in metals, which fits with the most widely accepted theory of cosmic evolution.
A network of 350 radio telescopes in South Africa’s Karoo desert is getting closer to detecting “cosmic dawn,” the period after the Big Bang when stars first ignited and galaxies began to bloom.
The Hydrogen Epoch of Reionization Array (HERA) team reports in a paper accepted for publication in The Astrophysical Journal that it has doubled the sensitivity of the array, which was already the most sensitive radio telescope in the world dedicated to exploring this unique period in the history of the universe.
While they have yet to detect radio emissions from the end of the cosmic dark ages, their findings do provide insights into the early universe’s star and galaxy composition. Their findings suggest that, unlike our galaxies today, early galaxies contained very few elements other than hydrogen and helium.
Early galaxies have to have been significantly different than the galaxies that we observe today in order for us not to have seen a signal. In particular, their X-ray characteristics have to have changed. Otherwise, we would have detected the signal we’re looking for.
Aaron Parsons
When the radio dishes are fully operational and calibrated, which should be this fall, the team hopes to create a 3D map of the evolution of ionized and neutral hydrogen bubbles from about 200 million years ago to about 1 billion years after the Big Bang. The map could reveal how early stars and galaxies differed from those we see today, as well as how the universe looked in its infancy.
“This is progressing toward a potentially revolutionary cosmological technique. There is so much information in the data once you narrow it down to the sensitivity you require” Joshua Dillon, the paper’s lead author and a research scientist in the Department of Astronomy at the University of California, Berkeley. “A 3D map of most of the luminous matter in the universe is the goal for the next 50 years or more.”
Other telescopes are looking into the early universe as well. The new James Webb Space Telescope (JWST) has imaged a galaxy that existed approximately 325 million years after the Big Bang. However, the JWST can only see the brightest galaxies that formed during the Reionization Epoch, not the smaller but far more numerous dwarf galaxies whose stars heated the intergalactic medium and ionized most of the hydrogen gas.
HERA seeks to detect radiation from the neutral hydrogen that filled the space between those early stars and galaxies, as well as to determine when that hydrogen ceased emitting or absorbing radio waves due to ionization.
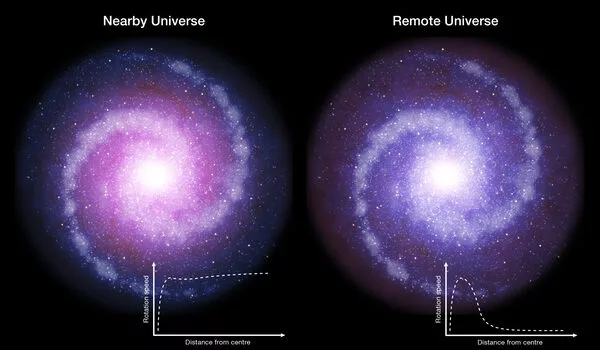
Because the HERA team has yet to detect these bubbles of ionized hydrogen within the cold hydrogen of the cosmic dark age, some theories of how stars evolved in the early universe are ruled out.
The data specifically show that the first stars, which may have formed around 200 million years after the Big Bang, contained few elements other than hydrogen and helium. This contrasts with the composition of today’s stars, which contain a variety of so-called metals, the astronomical term for elements heavier than helium ranging from lithium to uranium. The discovery fits with the current model for how stars and stellar explosions produce the majority of the other elements.
“Early galaxies have to have been significantly different than the galaxies that we observe today in order for us not to have seen a signal,” said Aaron Parsons, principal investigator for HERA and a UC Berkeley associate professor of astronomy. “In particular, their X-ray characteristics have to have changed. Otherwise, we would have detected the signal we’re looking for.”
The atomic composition of stars in the early universe determined how long it took for stars to form and heat the intergalactic medium. The high-energy radiation, primarily X-rays, produced by binary stars in which one has collapsed to a black hole or neutron star and is gradually devouring its companion is critical to this. Because there are few heavy elements, much of the companion’s mass is blown away rather than falling into the black hole, resulting in fewer X-rays and less heating of the surrounding region.
The new data fit the most popular theories of how stars and galaxies first formed after the Big Bang, but not others. Preliminary results from the first analysis of HERA data, reported a year ago, hinted that those alternatives – specifically, cold reionization – were unlikely.
“Our findings imply that the gas between galaxies was heated by X-rays even before reionization and as late as 450 million years after the Big Bang. These were most likely derived from binary systems in which one star is losing mass to a companion black hole “Dillon stated. “If that’s the case, those stars must have had very low’metallicity,’ that is, very few elements other than hydrogen and helium in comparison to our sun, which makes sense because we’re talking about a time in the universe before most of the other elements were formed.”
The Epoch of Reionization
The origin of the universe in the Big Bang 13.8 billion years ago produced a hot cauldron of energy and elementary particles that cooled for hundreds of thousands of years before protons and electrons combined to form atoms – primarily hydrogen and helium. Looking at the sky with sensitive telescopes, astronomers have mapped in detail the faint variations in temperature from this moment – what’s known as the cosmic microwave background – a mere 380,000 years after the Big Bang.
HERA and other experiments are focusing on a wavelength of light that neutral hydrogen absorbs and emits but ionized hydrogen does not. The hyperfine transition, which occurs when the electron and proton spins flip from parallel to antiparallel, produces the 21-centimeter line (a frequency of 1,420 megahertz). This radio frequency is not absorbed or emitted by ionized hydrogen, which has lost its sole electron.
Since the Epoch of Reionization, the 21-centimeter line has been red-shifted by the expansion of the universe to a wavelength 10 times as long – about 2 meters, or 6 feet. HERA’s rather simple antennas, a construct of chicken wire, PVC pipe, and telephone poles, are 14 meters across in order to collect and focus this radiation onto detectors.
“A chicken wire mesh is a mirror at two meters wavelength,” Dillon explained. “And all of the sophisticated stuff, so to speak, is in the supercomputer backend, as is all of the data analysis that follows.”
The new analysis is based on 94 nights of observing with approximately 40 antennas in 2017 and 2018 – phase 1 of the array. The preliminary analysis from last year was based on 18 nights of phase 1 observations.
The HERA team is working to improve the telescope’s calibration and data analysis in the hopes of seeing those bubbles in the early universe, which are about one millionth the intensity of radio noise in Earth’s neighborhood. It has not been easy to filter out the local radio noise in order to see the radiation from the early universe.