Three scientists jointly won this year’s Nobel Prize in physics on 04, October, for proving that tiny particles could retain a connection with each other even when separated, a phenomenon once doubted but now being explored for potential real-world applications such as encrypting information.
Frenchman Alain Aspect, American John F. Clauser, and Austrian Anton Zeilinger were cited by the Royal Swedish Academy of Sciences for experiments proving the “totally crazy” field of quantum entanglements to be all too real. They demonstrated that unseen particles, such as photons, can be linked, or “entangled,” with each other even when they are separated by large distances.
It all goes back to a feature of the universe that even baffled Albert Einstein and connects matter and light in a tangled, chaotic way. Bits of information or matter that used to be next to each other even though they are now separated have a connection or relationship—something that can conceivably help encrypt the information or even teleport. A Chinese satellite now demonstrates this and potentially lightning-fast quantum computers, still at the small and not quite useful stage, also rely on this entanglement. Others are even hoping to use it in the superconducting material.
“It’s so weird,” Aspect said of entanglement in a telephone call with the Nobel committee. “I am accepting in my mental images something which is totally crazy.”
Yet the trio’s experiments showed it happen in real life.
“Why this happens I haven’t the foggiest,” Clauser told The Associated Press during a Zoom interview in which he got the official call from the Swedish Academy several hours after friends and media informed him of his award. “I have no understanding of how it works but entanglement appears to be very real.”
His fellow winners also said they can’t explain the how and why behind this effect. But each did ever more intricate experiments that prove it just is.
Clauser, 79, was awarded his prize for a 1972 experiment, cobbled together with scavenged equipment, that helped settle a famous debate about quantum mechanics between Einstein and famed physicist Niels Bohr. Einstein described “a spooky action at a distance” that he thought would eventually be disproved.
“I was betting on Einstein,” Clauser said. “But unfortunately I was wrong and Einstein was wrong and Bohr was right.”
Aspect said Einstein may have been technically wrong, but deserves huge credit for raising the right question that led to experiments proving quantum entanglement.
Using entanglement you can transfer all the information which is carried by an object over to some other place where the object is, so to speak, reconstituted. He added that this only works for tiny particles. It is not like in the Star Trek films (where one is) transporting something, certainly not the person, over some distance.
Zeilinger
“Most people would assume that nature is made out of stuff distributed throughout space and time,” said Clauser, who while a high school student in the 1950s built a video game on a vacuum tube computer. “And that appears not to be the case.”
What the work shows is “parts of the universe—even those at great distances from each other—are connected,” said Johns Hopkins physicist N. Peter Armitage. “This is something so unintuitive and something so at odds with how we feel the world ‘should’ be.”
This hard-to-understand field started with thought experiments. But what in one sense is philosophical musings about the universe also holds hope for more secure and faster computers all based on entangled photons and matter that still interact no matter how distant.
“With my first experiments I was sometimes asked by the press what they were good for,” Zeilinger, 77, told reporters in Vienna. “And I said with pride: ‘It’s good for nothing. I’m doing this purely out of curiosity.'”
In quantum entanglement, establishing common information between two photons not near each other “allows us to do things like secret communication, in ways which weren’t possible to do before,” said David Haviland, chair of the Nobel Committee for Physics.
Quantum information “has broad and potential implications in areas such as secure information transfer, quantum computing, and sensing technology,” said Eva Olsson, a member of the Nobel committee. “Its predictions have opened doors to another world, and it has also shaken the very foundations of how we interpret measurements.”
The kind of secure communication used by China’s Micius satellite—as well as by some banks—is a “success story of quantum entanglement,” said Harun Siljak of Trinity College Dublin. By using one entangled particle to create an encryption key, it ensures that only the person with the other entangled particle can decode the message, and “the secret shared between these two sides is a proper secret,” Siljak said.
While quantum entanglement is “incredibly cool” security technologist Bruce Schneier, who teaches at Harvard, said it is fortifying an already secure part of information technology where other areas, including human factors and software, are more of a problem. He likened it to installing a side door with 25 locks on an otherwise insecure house.
At a news conference, Aspect said real-world applications like the satellite were “fantastic.”
“I think we have progressed toward quantum computing. I would not say that we are close,” the 75-year-old physicist said. “I don’t know if I will see it in my life. But I am an old man.”
Speaking by phone to a news conference after the announcement, the University of Vienna-based Zeilinger said he was “still kind of shocked” at hearing he had received the award. Clauser, Aspect and Zeilinger have figured in Nobel speculation for more than a decade. In 2010 they won the Wolf Prize in Israel, seen as a possible precursor to the Nobel.
The Nobel committee said Clauser developed quantum theories first put forward in the 1960s into a practical experiment. The aspect was able to close a loophole in those theories, while Zeilinger demonstrated a phenomenon called quantum teleportation that effectively allows information to be transmitted over distances.
“Using entanglement you can transfer all the information which is carried by an object over to some other place where the object is, so to speak, reconstituted,” Zeilinger said. He added that this only works for tiny particles. “It is not like in the Star Trek films (where one is) transporting something, certainly not the person, over some distance,” he said.
The prizes carry a cash award of 10 million Swedish kronor (nearly $900,000) and will be handed out on Dec. 10. The money comes from a bequest left by the prize’s creator, Swedish dynamite inventor Alfred Nobel, who died in 1895.
Nobel Committee press release: The Nobel Prize in Physics 2022
The Royal Swedish Academy of Sciences has decided to award the Nobel Prize in Physics 2022 to
Alain Aspect
Université Paris-Saclay and
École Polytechnique, Palaiseau, France
John F. Clauser
J.F. Clauser & Assoc., Walnut Creek, CA, U.S.
Anton Zeilinger
University of Vienna, Austria
“for experiments with entangled photons, establishing the violation of Bell inequalities and pioneering quantum information science”
Entangled states—from theory to technology
Alain Aspect, John Clauser and Anton Zeilinger have each conducted groundbreaking experiments using entangled quantum states, where two particles behave like a single unit even when they are separated. The ineffable effects of quantum mechanics are starting to find applications. There is now a large field of research that includes quantum computers, quantum networks, and secure quantum encrypted communication.
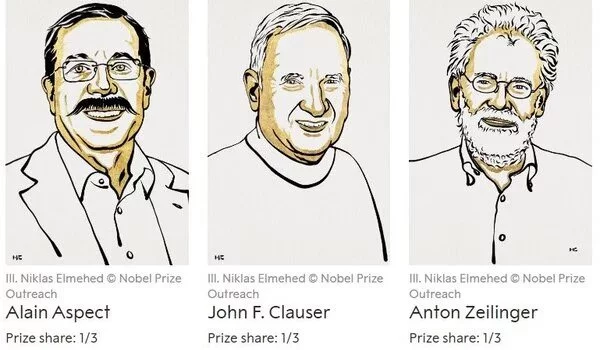
For a long time, the question was whether the correlation was because the particles in an entangled pair contained hidden variables, instructions that tell them which result they should give in an experiment. In the 1960s, John Stewart Bell developed the mathematical inequality that is named after him. This states that if there are hidden variables, the correlation between the results of a large number of measurements will never exceed a certain value. However, quantum mechanics predicts that a certain type of experiment will violate Bell’s inequality, thus resulting in a stronger correlation than would otherwise be possible.
John Clauser developed John Bell’s ideas, leading to a practical experiment. When he took the measurements, they supported quantum mechanics by clearly violating a Bell inequality. This means that quantum mechanics cannot be replaced by a theory that uses hidden variables.
Some loopholes remained after John Clauser’s experiment. Alain Aspect developed the setup, using it in a way that closed an important loophole. He was able to switch the measurement settings after an entangled pair had left its source, so the setting that existed when they were emitted could not affect the result.
Using refined tools and long series of experiments, Anton Zeilinger started to use entangled quantum states. Among other things, his research group has demonstrated a phenomenon called quantum teleportation, which makes it possible to move a quantum state from one particle to one at a distance.
How entanglement has become a powerful tool
Using groundbreaking experiments, Alain Aspect, John Clauser, and Anton Zeilinger have demonstrated the potential to investigate and control particles that are in entangled states. What happens to one particle in an entangled pair determines what happens to the other, even if they are really too far apart to affect each other. The laureates’ development of experimental tools has laid the foundation for a new era of quantum technology.
The fundamentals of quantum mechanics are not just theoretical or philosophical issues. Intense research and development are underway to utilize the special properties of individual particle systems to construct quantum computers, improve measurements, build quantum networks and establish secure quantum encrypted communication.
Many applications rest upon how quantum mechanics allow two or more particles to exist in a shared state, regardless of how far apart they are. This is called entanglement and has been one of the most debated elements of quantum mechanics ever since the theory was formulated. Albert Einstein talked about spooky action at a distance and Erwin Schrödinger said it was quantum mechanics’ most important trait.
This year’s laureates have explored these entangled quantum states, and their experiments laid the foundation of the revolution currently underway in quantum technology.
Far from everyday experience
When two particles are in entangled quantum states, someone who measures a property of one particle can immediately determine the result of an equivalent measurement on the other particle, without needing to check.
What makes quantum mechanics so special is that its equivalents to the balls have no determined states until they are measured. It is as if both the balls are grey, right up until someone looks at one of them. Then, it can randomly take either all the black the pair of balls has access to, or can show itself to be white. The other ball immediately turns the opposite color.
But how is it possible to know that the balls did not each have a set color at the beginning? Even if they appeared grey, perhaps they had a hidden label inside, saying which color they should turn when someone looks at them.
Does color exist when no one is watching?
Quantum mechanics’ entangled pairs can be compared to a machine that throws out balls of opposite colors in opposite directions. When Bob catches a ball and sees that it is black, he immediately knows that Alice has caught a white one. In a theory that uses hidden variables, the balls had always contained hidden information about what color to show. However, quantum mechanics says that the balls were grey until someone looked at them, when one randomly turned white and the other black. Bell inequalities show that there are experiments that can differentiate between these cases. Such experiments have proven that quantum mechanics’ description is correct.
An important part of the research being rewarded with this year’s Nobel Prize in Physics is a theoretical insight called Bell inequalities. Bell inequalities make it possible to differentiate between quantum mechanics’ indeterminacy and an alternative description using secret instructions, or hidden variables. Experiments have shown that nature behaves as predicted by quantum mechanics. The balls are grey, with no secret information, and chance determines which becomes black and which becomes white in an experiment.
Quantum mechanics’ most important resource
Entangled quantum states hold the potential for new ways of storing, transferring, and processing information.
Interesting things happen if the particles in an entangled pair travel in opposite directions and one of them then meets the third particle in such a manner that they become entangled. They then enter a new shared state. The third particle loses its identity, but its original properties have now been transferred to the solo particle from the original pair. This way of transferring an unknown quantum state from one particle to another is called quantum teleportation. This type of experiment was first conducted in 1997 by Anton Zeilinger and his colleagues.
Remarkably, quantum teleportation is the only way to transfer quantum information from one system to another without losing any part of it. It is absolutely impossible to measure all the properties of a quantum system and then send the information to a recipient who wants to reconstruct the system. This is because a quantum system can contain several versions of every property simultaneously, where each version has a certain probability of appearing during a measurement. As soon as the measurement is conducted, only one version remains, namely the one that was read by the measuring instrument. The others have disappeared and it is impossible to ever know anything about them. However, entirely unknown quantum properties can be transferred using quantum teleportation and appear intact in another particle, but at the price of them being destroyed in the original particle.
Once this had been shown experimentally, the next step was to use two pairs of entangled particles. If one particle from each pair are brought together in a particular way, the undisturbed particles in each pair can become entangled despite never having been in contact with each other. This entanglement swapping was first demonstrated in 1998 by Anton Zeilinger’s research group.
Entangled pairs of photons, particles of light, can be sent in opposite directions through optical fibers and function as signals in a quantum network. Entanglement between two pairs makes it possible to extend the distances between the nodes in such a network. There is a limit to the distance that photons can be sent through optical fiber before they are absorbed or lose their properties. Ordinary light signals can be amplified along the way, but this does not work with entangled pairs. An amplifier has to capture and measure the light, which breaks the entanglement. However, entanglement swapping means it is possible to send the original state further, thereby transferring it over longer distances than had otherwise been possible.
Entangled particles that never met
Two pairs of entangled particles are emitted from different sources. One particle from each pair is brought together in a special way that entangles them. The two other particles (1 and 4 in the diagram) are then also entangled. In this way, two particles that have never been in contact can become entangled.
From paradox to inequality
This progress rests on many years of development. It started with the mind-boggling insight that quantum mechanics allows a single quantum system to be divided up into parts that are separated from each other but which still act as a single unit.
This goes against all the usual ideas about cause and effect and the nature of reality. How can something be influenced by an event occurring somewhere else without being reached by some form of signal from it? A signal cannot travel faster than light—but in quantum mechanics, there does not seem to be any need for a signal to connect the different parts of an extended system.
Albert Einstein regarded this as unfeasible and examined this phenomenon, along with his colleagues Boris Podolsky and Nathan Rosen. They presented their reasoning in 1935: quantum mechanics does not appear to provide a complete description of reality. This has come to be called the EPR paradox, after the researchers’ initials.
The question was whether there could be a more complete description of the world, where quantum mechanics is just one part. This could, for example, work through particles always carrying hidden information about what they will show as the result of an experiment. All the measurements then show the properties that exist exactly where the measurements are conducted. This type of information is often called local hidden variables.
The Northern Irish physicist John Stewart Bell (1928–1990), who worked at CERN, the European particle physics laboratory, took a closer look at the problem. He discovered that there is a type of experiment that can determine whether the world is purely quantum mechanical, or whether there could be another description with hidden variables. If his experiment is repeated many times, all theories with hidden variables show a correlation between the results that must be lower than, or at most equal to, a specific value. This is called Bell’s inequality.
However, quantum mechanics can violate this inequality. It predicts higher values for the correlation between the results than is possible through hidden variables.
John Clauser became interested in the fundamentals of quantum mechanics as a student in the 1960s. He could not shake of John Bell’s idea once he had read about it and, eventually, he and three other researchers were able to present a proposal for a realistic type of experiment that can be used to test a Bell inequality.
The experiment involves sending a pair of entangled particles in opposite directions. In practice, photons that have a property called polarisation are used. When the particles have emitted the direction of the polarization is undetermined, and all that is certain is that the particles have parallel polarization. This can be investigated using a filter that allows polarisation that is oriented in a particular direction (see figure Experimenting with Bell inequalities). This is the effect used in many sunglasses, which block light that has been polarised in a certain plane, for example by reflecting water.
If both the particles in the experiment are sent towards filters that are oriented in the same plane, such as vertically, and one slips through—then the other one will also go through. If they are at right angles to each other, one will be stopped while the other will go through. The trick is to measure with the filters set in different directions at skewed angles, as then the results can vary: sometimes both slip through, sometimes just one, and sometimes none. How often both particles get through the filter depends on the angle between the filters.
Quantum mechanics leads to a correlation between measurements. The likelihood of one particle getting through depends on the angle of the filter that tested its partner’s polarisation on the opposite side of the experimental setup. This means that the results of both measurements, at some angles, violate a Bell inequality and have a stronger correlation than they would if the results were governed by hidden variables and were already predetermined when the particles were emitted.
Violated inequality
John Clauser immediately started working on conducting this experiment. He built an apparatus that emitted two entangled photons at a time, each towards a filter that tested their polarization. In 1972, along with doctoral student Stuart Freedman (1944–2012), he was able to show a result that was a clear violation of a Bell inequality and agreed with the predictions of quantum mechanics.
In the years that followed, John Clauser and other physicists continued discussing the experiment and its limitations. One of these was that the experiment was generally efficient, both when it came to producing and capturing particles. The measurement was also pre-set, with the filters at fixed angles. There were therefore loopholes, where an observer could question the results: what if the experimental setup in some way selected the particles that happened to have a strong correlation, and did not detect the others? If so, the particles could still be carrying hidden information.
Eliminating this particular loophole was difficult, because entwined quantum states are so fragile and difficult to manage; it is necessary to deal with individual photons. French doctoral student Alain Aspect was not intimidated, and built a new version of the setup that he refined over several iterations. In his experiment, he could register the photons that passed through the filter and those that did not. This meant more photons were detected and the measurements were better.
In the final variant of his tests, he was also able to steer photons towards two different filters that were set at different angles. The finesse was a mechanism that switched the direction of the entangled photons after they had been created and emitted from their source. The filters were just six meters away, so the switch needed to occur in a few billionths of a second. If information about which filter the photon would arrive at influenced how it was emitted from the source, it would not be arriving at that filter. Nor could information about the filters on one side of the experiment reach the other side and affect the result of the measurement there.
In this way, Alain Aspect closed an important loophole and provided a very clear result: quantum mechanics is correct and there are no hidden variables.
The era of quantum information
The era of quantum information These and similar experiments laid the foundation for the current intense research in quantum information science. Being able to manipulate and manage quantum states and all their layers of properties gives us access to tools with unexpected potential. This is the basis for quantum computation, the transfer and storage of quantum information, and algorithms for quantum encryption. Systems with more than two particles, all of which are entangled, are now in use, which Anton Zeilinger and his colleagues were the first to explore.
Experimenting with Bell inequalities
Anton Zeilinger later conducted more tests of Bell inequalities. He created entangled pairs of photons by shining a laser on a special crystal, and used random numbers to shift between measurement settings. One experiment used signals from distant galaxies to control the filters and ensure the signals could not affect each other.
These increasingly refined tools bring realistic applications ever closer. Entangled quantum states have now been demonstrated between photons that have been sent through tens of kilometres of optical fibre, and between a satellite and a station on the ground. In a short time, researchers around the world have found many new ways to utilize the most powerful property of quantum mechanics.