An international team of researchers, led by the PRISMA+ Cluster of Excellence at Johannes Gutenberg University Mainz (JGU) and the Helmholtz Institute Mainz (HIM), has published full data on the search for dark matter utilizing a global network of optical magnetometers for the first time. According to the scientists, dark matter fields should provide a distinct signal pattern that may be discovered using correlated observations from numerous GNOME network locations.
Data analysis from a one-month continuous GNOME operation has failed to produce a relevant indication. However, as the researchers explain in the journal Nature Physics, the measurement allows them to set restrictions on the properties of dark matter.
GNOME is an acronym that stands for Global Network of Optical Magnetometers for Exotic Physics Research. Magnetometers in Germany, Serbia, Poland, Israel, South Korea, China, Australia, and the United States are powering it. The researchers hope to use GNOME to further the search for dark matter, which is one of the most fascinating issues in fundamental physics in the twenty-first century. After all, it has long been known that dark matter may best explain many intriguing astronomical findings, such as the rotation speed of stars in galaxies or the spectrum of cosmic background radiation.
If such a wall collides with the Earth, it is progressively recognized by the GNOME network and can generate brief characteristic signal patterns in the magnetometers. Even more, the signals are connected in specific ways based on how rapidly the wall moves and when it reaches each point.
Dr. Arne Wickenbrock
“Extremely light bosonic particles are considered one of the most promising candidates for dark matter today. These include so-called axion-like particles — ALPs for short,” said Professor Dr. Dmitry Budker, professor at PRISMA+ and at HIM, an institutional collaboration of Johannes Gutenberg University Mainz and the GSI Helmholtzzentrum für Schwerionenforschung in Darmstadt.
“They can alternatively be thought of as a classical field vibrating at a specific frequency. A feature of such bosonic fields is their ability to build patterns and structures, according to one plausible theoretical scenario. As a result, the quantity of dark matter could be concentrated in a variety of locations, such as discrete domain walls smaller than a galaxy but much larger than Earth.”
“If such a wall collides with the Earth, it is progressively recognized by the GNOME network and can generate brief characteristic signal patterns in the magnetometers,” one of the study’s co-authors, Dr. Arne Wickenbrock, noted. “Even more, the signals are connected in specific ways based on how rapidly the wall moves and when it reaches each point.”
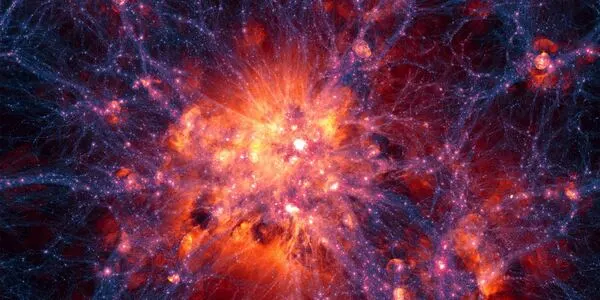
Meanwhile, the network is made up of 14 magnetometers spread across eight countries, with nine of them providing data for the current analysis. The measurement principle is based on dark matter interacting with the nuclear spins of the magnetometer’s atoms. The atoms are activated using a laser at a precise frequency, which causes the nuclear spins to be oriented in one direction. This direction can be disturbed by a possible dark matter field, which is detectable.
Figuratively speaking, one can imagine that the atoms in the magnetometer initially dance around in confusion, as clarified by Hector Masia-Roig, a doctoral student in the Budker group and also an author of the current study. “When they ‘hear’ the right frequency of laser light, they all spin together. Dark matter particles can throw the dancing atoms out of balance. We can measure this perturbation very precisely.”
The network of magnetometers is now crucial: The dancing atoms in all stations are gradually disturbed as the Earth travels through a spatially constrained wall of dark matter. One of these stations is housed in a laboratory at Mainz’s Helmholtz Institute. “Only by matching the signals from all the stations can we determine what caused the disturbance,” Masia-Roig explained. “In the case of the dancing atoms, this means that if we compare the measurement findings from all the stations, we can determine whether it was just one bold dancer dancing out of step or a worldwide dark matter disturbance.”
The current study evaluates data from a one-month continuous running of GNOME. The result: No statistically significant signals were found in the examined mass range of one femtoelectronvolt (feV) to 100,000 feV. In turn, this means that the researchers can limit down the range in which such signals could conceivably be located even further than before. This is an important conclusion for scenarios that rely on discrete dark matter barriers, “even if we have not yet been able to detect such a domain wall with our global ring search,” stated Joseph Smiga, another Mainz PhD student and research author.
The GNOME collaboration’s future work will concentrate on enhancing both the magnetometers and the data analysis. Continuous operation, in particular, should be more stable. This is necessary in order to search for signals that endure more than an hour. Furthermore, the previous alkali atoms in the magnetometers will be replaced with noble gases. The researchers expect Advanced GNOME to produce in much improved sensitivity for future studies in the search for ALPs and dark matter.