Researchers have developed a tool to better understand what might ‘switch on’ bacterial immune defenses by understanding the function of an elusive small DNA in bacteria.
Retrons have perplexed researchers who have simply wanted to know what these bacterial DNA sequences did since they were discovered in the 1980s. EMBL researchers have discovered that some retrons encode toxin proteins that are rendered inactive by a small DNA fragment. When a bacterial virus (phage) attacks bacteria, the toxin is released by the small DNA.
“Bacterial chromosomes contain hundreds of different toxin/antitoxin systems of unknown function that might be leveraged to inhibit phages, and our findings provide an approach to understand how they could do that,” said Nassos Typas, a group leader in the Genome Biology Unit and a co-chair of EMBL’s Microbial Ecosystems and Infection Biology transversal themes. His group has just reported its latest findings in Nature.
Simply put, retrons contain a reverse transcriptase enzyme that uses small RNA as a template to produce multicopy single-stranded DNA (msDNA). Although scientists knew how this msDNA is produced in many bacteria, its function and role in the cell remained a mystery until June 2020, when the Typas group and the Sorek group from Israel’s Weizmann Institute of Science published their independent studies in an open-access preprint repository.
We discovered that msDNA and reverse transcriptase form a new class of antitoxins. But we were still curious about the ‘switch’ that would naturally activate this growth inhibition complex.
Jacob Bobonis
“For more than 30 years, we’d had no clue why bacteria have retrons because no phenotypes had been associated with cells lacking retrons or msDNA,” said Jacob Bobonis, the paper’s lead author, who completed his Ph.D. in the Typas group.
However, new information was discovered when a previous member of the Typas group discovered an important clue — a phenotype. They discovered that the pathogenic bacterium Salmonella cannot grow at lower temperatures unless it produces msDNA. The group then collaborated with Helene Andrews-Polymenis’ lab at Texas A&M University and her then-postdoc Johanna Elfenbein, who is now a PI at the University of Wisconsin. They discovered that Salmonella cells that were unable to produce msDNA were also sensitive to a lack of oxygen, preventing them from colonizing a cow’s gut.
While these phenotypes did not demonstrate the retrons’ unique immune defense abilities, they did provide scientists with a starting point for further research into the retrons.
“We quickly realised that retrons, while more complicated, looked very similar to other systems in bacteria called toxin/antitoxin systems,” Bobonis explained.
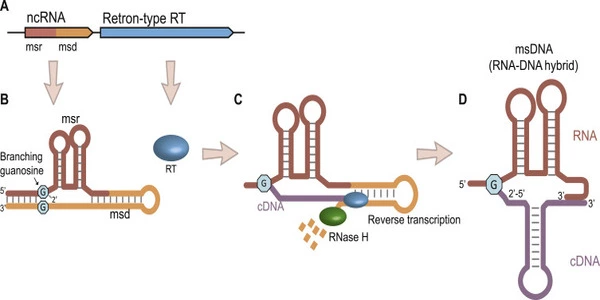
Hundreds of toxin/antitoxin systems are found in the genomes of many bacteria. One gene encodes a poisonous protein (toxin) that inhibits bacterial growth, but the antidote (antitoxin) is found right next to the ‘poisonous’ gene. Bacteria thrive while the two coexist. However, if the antidote is lost, the poison becomes active and inhibits their growth.
“In our case, we have the retron reverse transcriptase, which makes msDNA, and if we delete it, the ‘toxin’ is activated,” Bobonis explained. “We discovered that msDNA and reverse transcriptase form a new class of antitoxins. But we were still curious about the ‘switch’ that would naturally activate this growth inhibition complex.”
These natural switches (triggers) for all chromosomal toxin/antitoxin systems have remained elusive for decades. The EMBL team decided to look into whether individual genes could function as switches. They overexpressed thousands of bacterial genes one by one in EMBL labs using robotic setups to see if they could trigger the toxin to inhibit the bacteria.
Finally, using genetics, proteomics, and bioinformatics, and with the assistance of other EMBL teams (the Savitski, Zeller, and Bateman research groups), they figured out how viral proteins can both activate and block these systems. They discovered that retrons can prevent viral invasion on a single cell level.
“Imagine you have 10 bacteria, and a virus goes in and infects just one of them. The virus replicates itself hundreds of times, eventually breaking the cell so that virus spills over from the infected cell, and goes on to infect the other nine cells (or more if bacteria have duplicated in the meantime). In that case, the bacterial population is killed,” Bobonis explained. “In a cell where the retron is switched on by the virus, the initial infected cell withers, but so does the virus, as it needs the bacterium’s machinery to replicate. Without the initially infected bacterium, the virus falters, and retrons have protected the rest of the population.”
Scientists are investigating the use of phages to treat bacterial infections in humans. This is known as phage therapy, and research in this area has increased dramatically in recent years as antibiotics become less effective due to resistance issues. This fundamental research contributes to the body of knowledge, which will aid in the advancement of that work.
The genetics-based approach refined by the EMBL scientists, Toxin Activation/Inhibition Conjugation (TAC/TIC), is an important outcome of this research. Other scientists can now zero in on what causes the thousands of other unidentified toxin/antitoxin systems. At the heart of EMBL’s new Microbial Ecosystems, transversal theme is tapping into the vast diversity of microbial functions and uncovering new mechanisms that lie behind the interactions of microbes with their environment, including their predators.
“Because these are bacteria’s internal suicide systems, knowing the trigger switches allow us to design artificial toxin triggers to externally activate the toxin and kill the cell,” Typas explained. “Such new strategies are urgently needed as effective antibiotics become scarce to treat antimicrobial-resistant pathogens.” The Infection Biology transversal theme at EMBL aims to better understand antimicrobial resistance and find new ways to curb, prevent, reverse, or bypass it.