Understanding the interaction between magnetism and highorder topology in a quantum material is currently one of the most difficult research fields in materials science, with future spintronics applications as the goal. Among the exotic new quantum phases in three-dimensional (3D) magnetic topological insulators, the breaking of time-reversal symmetry (TRS) induced by magnetic order is a key element that enables many topological phase transitions; TRS breaking is the basis of the quantum anomalous Hall effect and the topological magnetoelectric (TME) effect.
Recent review work on magnetic topological materials proposes a novel theoretical notion that combines magnetism with topology. Andrei Bernevig, Princeton University, USA, Haim Beidenkopf, Weizmann Institute of Science, Israel, and Claudia Felser, Max Planck Institute for Chemical Physics of Solids, Dresden, Germany, introduce a new theoretical concept that combines magnetism and topology in a new review paper on magnetic topological materials.
It discovers and examines prospective novel magnetic topological materials, and discusses their potential future uses in spin and quantum electronics, as well as materials for efficient energy conversion. The review addresses the relationship between topology, symmetry, and magnetism at a level appropriate for graduate students in physics, chemistry, and materials science with a basic understanding of condensed matter physics.
If we can create a magnetic catalyst for water splitting, we might be able to adjust the catalytic characteristics with an external field, allowing us to turn on and off catalysis. My main goal for the future is to design a material that exhibits a high temperature quantum anomalous Hall via quantum confinement of a magnetic Weyl semimetal, and its integration into quantum devices.
Haim Beidenkopf
Magnetic topological materials are a type of substance whose properties are heavily impacted by the topology of the electronic wavefunctions in conjunction with their spin configuration. Topology is a straightforward concept that deals with the surfaces of objects. A mathematical structure’s topology is identical if it is preserved during continuous deformation. A pancake has the same topology as a cube, a donut has the same topology as a coffee cup, and a pretzel has the same topology as a three-holed board. Adding spin provides additional structure – a new degree of freedom – allowing the realization of hitherto unknown states of matter in non-magnetic materials.
Magnetic topological materials can enable chiral electron and spin channels and can be used for a variety of applications ranging from information storage to control of dissipationless spin and charge transport to enormous responses to external stimuli such as temperature and light.
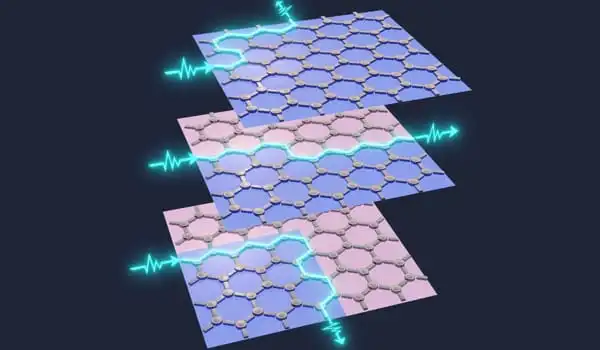
The review summarizes theoretical and experimental advances in the field of magnetic topological materials, beginning with the theoretical prediction of the Quantum Anomalous Hall Effect without Landau levels and progressing to the recent discoveries of magnetic Weyl semimetals and antiferromagnetic topological insulators. The most recent theoretical advances, which resulted in the tabulation of all magnetic symmetry group representations and topology, are discussed.
As a result, all known magnetic materials, including those yet to be discovered, can be fully defined by their topological features. It is simple to identify materials for a certain technical purpose (e.g., Quantum Anomalous Hall). Magnetic topological materials with magnetic transition temperatures above room temperature can be identified and, if necessary, designed for classical applications such as thermoelectric devices, Hall sensors, or efficient catalysts using this approach, but they are also useful for quantum applications at low temperatures such as computing and sensing.
“The realization of the QAHE at room temperature would be revolutionary,” says Andrei Bernevig, “overcoming the limitations of many data-based technologies, which are affected by power losses from Joule heating,” and his colleague Stuart Parkin, Max PIanck Institute of Microstructure Physics, Halle, Germany, “can imagine how the novel properties of this new class of magnetic materials can pave the way to new generations of low energy consuming quantum electronic and spherical materials.”
Claudia Felser, MPI CPfS, is particularly enthusiastic about their prospective uses in chemistry. “If we can create a magnetic catalyst for water splitting, we might be able to adjust the catalytic characteristics with an external field, allowing us to turn on and off catalysis,” she explains. The quantum computer is perhaps the most exciting direction in science today, according to Haim Beidenkopf: “My main goal for the future is to design a material that exhibits a high temperature quantum anomalous Hall via quantum confinement of a magnetic Weyl semimetal, and its integration into quantum devices.” Magnetic topological materials have definitely had and will continue to have an impact in both the scientific and technological sectors.